Role of ureides in source-to-sink transport of photoassimilates in non-fixing soybean - PubMed
- ️Wed Jan 01 2020
Role of ureides in source-to-sink transport of photoassimilates in non-fixing soybean
Sandi Win Thu et al. J Exp Bot. 2020.
Abstract
Nitrogen (N)-fixing soybean plants use the ureides allantoin and allantoic acid as major long-distance transport forms of N, but in non-fixing, non-nodulated plants amino acids mainly serve in source-to-sink N allocation. However, some ureides are still synthesized in roots of non-fixing soybean, and our study addresses the role of ureide transport processes in those plants. In previous work, legume ureide permeases (UPSs) were identified that are involved in cellular import of allantoin and allantoic acid. Here, UPS1 from common bean was expressed in the soybean phloem, which resulted in enhanced source-to-sink transport of ureides in the transgenic plants. This was accompanied by increased ureide synthesis and elevated allantoin and allantoic acid root-to-sink transport. Interestingly, amino acid assimilation, xylem transport, and phloem partitioning to sinks were also strongly up-regulated. In addition, photosynthesis and sucrose phloem transport were improved in the transgenic plants. These combined changes in source physiology and assimilate partitioning resulted in increased vegetative growth and improved seed numbers. Overall, the results support that ureide transport processes in non-fixing plants affect source N and carbon acquisition and assimilation as well as source-to-sink translocation of N and carbon assimilates with consequences for plant growth and seed development.
Keywords: Amino acid assimilation; legume; nitrogen and carbon metabolism; phloem loading; photoassimilate partitioning; seed development; source-to-sink transport; soybean; ureide transporter function.
© The Author(s) 2020. Published by Oxford University Press on behalf of the Society for Experimental Biology. All rights reserved. For permissions, please email: journals.permissions@oup.com.
Figures
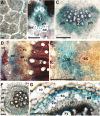
PvUPS1 promoter–GUS analysis in soybean. (A–C) Source leaves. (A) GUS staining is evident in the leaf vasculature of (B) minor and (C) major veins, including the phloem, bundle sheath cells, and vascular parenchyma. Scale bars=1 mm in (A), 50 μm in (B) and (C). (D and E) Stem cross-sections. Staining is seen in the phloem and surrounding cells. Scale bars=250 μm. (F and G) Root cross-sections. GUS expression is reported in the endodermis, pericycle, phloem, and vascular parenchyma. Scale bars=50 μm. Bundle sheath cells, bs; cambium, ca; cortex, co; endodermis, en; pericycle, pc; phloem fiber cap, fc; parenchyma, pa; phloem, ph; primary phloem, pp; secondary phloem, sp; sclerenchyma cells, sc; xylem, xy.
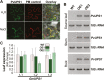
Membrane localization of common bean PvUPS1 and analysis of ureide transporter transcript levels in transgenic soybean plants expressing PvUPS1 under control of the PvUPS1 promoter (see Fig. 1). (A) Localization of GFP–PvUPS1 fusion proteins in leaf epidermal cells of Nicotiana benthamiana. The top row shows turgid cells. The bottom row shows NaCl-treated, plasmolyzed cells. The aquaporin (AtPIP2A)–mCherry fusion protein was used as control for plasma membrane localization. Plasma membrane co-localization of GFP–PvUPS1 and AtPIP2A–mCherry is shown in the overlay image. (B) Expression analysis of PvUPS1 in source leaves, stems, and roots of soybean UPS1 overexpressor lines OE1 and OE2 and wild-type plants (WT) using RT–PCR. (C) Expression of the three soybean ureide transporter genes GmUPS1-1, 1-2, and 1-3 in source leaves of the OE lines using qPCR (quantitative real-time PCR). Data derive from at least four biological replicates (n ≥4) and were tested by Student’s t-test. Error bars depict ±SD.
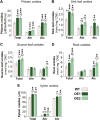
Analyses of total ureides, allantoin, and allantoic acid in (A) leaf phloem exudates (n=5), (B) sink leaves (n ≥3), (C) source leaves (n ≥3), (D) roots (n=3), and (E) xylem (n ≥3) of UPS1-overexpressing soybean lines (OE1 and OE2) and wild-type (WT) plants. Allantoin, Aln; allantoic acid, Alc. Data were tested by one-way ANOVA. Error bars depict ±SD, and asterisks indicate small, moderate, and large statistically significant changes from the WT (*P≤0.05, **P≤0.01, ***P≤0.001). Numbers above columns show the percentage change in UPS1-OE lines compared with the WT.
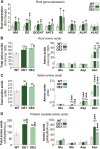
Effects of UPS1 overexpression on root nitrogen metabolism and root-to-shoot-to-sink amino acid transport. Results are shown for UPS1-overexpressing soybean lines OE1 and OE2 and wild-type (WT) plants, and are presented as means ±SD, with SD indicated by error bars. Data were tested by Student’s t-test (A) or one-way ANOVA (B–D). Small, moderate, and large statistically significant changes from the WT are marked with asterisks (*P≤0.05, **P≤0.01, ***P≤0.001). Numbers above columns show the percentage change in UPS1-OE lines compared with the WT. (A) Expression of genes related to N assimilation, and amino acid and ureide synthesis was analyzed by qPCR in lines OE1 and OE2 and WT plants (n ≥6). The analyses included genes coding for nitrate reductase (NIA), and genes related to amino acid assimilation (GS, glutamine synthetase; GOGAT, 2-oxoglutarate amino transferase), synthesis of aspartate (AAT, aspartate aminotransferase) and asparagine (AS, asparagine synthetase), as well as synthesis of allantoin (HIUH, hydroxyisourate hydrolase) and allantoic acid (ALN, allantoinases). For gene accessions, primer sequences, and references, see Supplementary Table S1. Shown is the fold change in gene expression in UPS1-OE lines compared with the WT and relative to SKIP16 expression. (B–D) Analysis of total free amino acids as well as the most abundant amino acids Glu, Gln, Asp, and Asn. (B) Root, (C) xylem sap, and (E) leaf phloem exudate amino acids. Results are from at least four biological repeats (n ≥4).
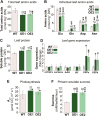
Effect of UPS1 overexpression on leaf N status, C fixation, and source-to-sink sucrose partitioning. Results are shown for UPS1-overexpressing soybean lines OE1 and OE2 and wild-type (WT) plants, and are presented as means ±SD, with SD indicated by error bars. Data were tested by one-way ANOVA (A–C, E, F) or Student’s t-test (D). Small, moderate, and large statistically significant changes from the WT are marked with asterisks (*P≤0.05, **P≤0.01, ***P≤0.001). Numbers above columns show the percentage change in UPS1-OE lines compared with the WT. (A) Total free amino acid levels in source leaves (n ≥4). (B) Leaf levels of the most abundant amino acids Glu, Gln, Asp, and Asn (n ≥4). (C) Leaf soluble protein (n ≥4). (D) Expression analysis of leaf genes related to amino acid and ureide catabolism, and synthesis of vegetative storage proteins by qPCR. The analyses included genes coding for asparaginases (ASPGB1a and ASPGB1b), allantoate amidohydrolase (AAH), ubiquitous urease (UU), and vegetative storage proteins α and β (VSPa and VSPb) (n ≥4). For gene accessions, primer sequences, and references, see Supplementary Table S1. Shown is the fold change in gene expression in soybean lines overexpressing UPS1 (OE) compared with the WT and relative to SKIP16 expression. (E) Net CO2 assimilation rate (n ≥4). (F) Sucrose levels in leaf phloem exudates (n=5).
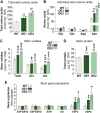
Effects of UPS1 overexpression on N storage in the stem. Results are shown for UPS1-overexpressing soybean lines OE1 and OE2 and wild-type (WT) plants, and are presented as means ±SD, with SD indicated by error bars. Data were tested by one-way ANOVA (A–D) or Student’s t-test (E). Small, moderate, and large statistically significant changes from the WT are marked with asterisks (*P≤0.05, **P≤0.01, ***P≤0.001). Numbers above columns show the percentage change in UPS1-OE lines compared with the WT. (A) Total free amino acid levels in stem (n ≥3). (B) Stem levels of the most abundant amino acids Glu, Gln, Asp, and Asn (n ≥3). (C) Stem levels of total ureides, allantoin (Aln), and allantoic acid (Alc) (n=5). (D) Stem soluble protein (n ≥3). (E) Expression analysis of genes related to amino acid and ureide catabolism, and synthesis of vegetative storage protein in stem. qPCR was performed for genes encoding asparaginases (ASPGB1a and ASPGB1b), allantoate amidohydrolase (AAH), ubiquitous urease (UU), and vegetative storage proteins α and β (VSPa and VSPb) (n ≥4).For gene accessions, primer sequences, and references see Supplementary Table S1. Shown is the fold change in gene expression in UPS1-OE lines compared with the WT and relative to SKIP16 expression.
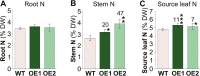
Analysis of elemental N in (A) roots, (B) stems, and (C) source leaves. Shown is the percentage (%) of N in dried tissues of UPS1-overexpressing soybean lines (OE1 and OE2) and wild-type (WT) plants (n=4). Data were tested by Student’s t-test. Error bars depict ±SD, and asterisks indicate small, moderate, and large statistically significant changes from the WT (*P≤0.05, **P≤0.01, ***P≤0.001). Numbers above columns show the percentage change in UPS1-OE lines compared with the WT.
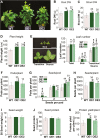
Effects of UPS1 overexpression on plant growth and seed development. Results are shown for 36-day-old UPS1-overexpressing soybean lines OE1 and OE2 and wild-type (WT) plants, and are presented as means ±SD, with SD indicated by error bars. Data were tested by one-way ANOVA (B and C) or Student’s t-test (D–K). Small, moderate, and large statistically significant changes from the WT are marked with asterisks (*P≤0.05, **P≤0.01, ***P≤0.001). Numbers above columns show the percentage change in UPS1-OE lines compared with the WT. (A) Growth phenotype of UPS1-OE and WT plants. (B) Root and (C) shoot biomass (DW; n ≥8). (D) Plant height. (E) Analysis of total leaf number and total number of sink leaves as well as transition and source leaves (n ≥9). The image shows leaves that were classified as sink, sink-to-source transition, and source leaves. Sink leaves were defined as new, developing, and folded leaves. The unfolded leaves included expanding leaves that transition from sink to source (i.e. transition leaves) as well as fully expanded source leaves. Scale bars=5 cm. (F) Total number of pods per plant at desiccation/harvest (n ≥9). (G) Distribution of one-, two-, and three-seeded pods (n ≥9). (H) Total number of seeds per plant at desiccation (n ≥9). (I) Fifty-seed weight at desiccation (n ≥9 plants with five measurements per plant). (J) Amount of soluble protein per dry seed (n ≥9). (K) Total seed protein yield per plant (n ≥9).
Similar articles
-
Lu MZ, Carter AM, Tegeder M. Lu MZ, et al. J Plant Physiol. 2022 Feb;269:153613. doi: 10.1016/j.jplph.2021.153613. Epub 2021 Dec 28. J Plant Physiol. 2022. PMID: 35033961
-
Tegeder M. Tegeder M. J Exp Bot. 2014 Apr;65(7):1865-78. doi: 10.1093/jxb/eru012. Epub 2014 Jan 31. J Exp Bot. 2014. PMID: 24489071 Review.
-
Lu MZ, Snyder R, Grant J, Tegeder M. Lu MZ, et al. Plant J. 2020 Jan;101(1):217-236. doi: 10.1111/tpj.14533. Epub 2019 Sep 14. Plant J. 2020. PMID: 31520495
-
PvUPS1 plays a role in source-sink transport of allantoin in French bean (Phaseolus vulgaris).
Pélissier HC, Tegeder M. Pélissier HC, et al. Funct Plant Biol. 2007 May;34(4):282-291. doi: 10.1071/FP06277. Funct Plant Biol. 2007. PMID: 32689354
-
Early signaling, synthesis, transport and metabolism of ureides.
Baral B, Teixeira da Silva JA, Izaguirre-Mayoral ML. Baral B, et al. J Plant Physiol. 2016 Apr 1;193:97-109. doi: 10.1016/j.jplph.2016.01.013. Epub 2016 Feb 22. J Plant Physiol. 2016. PMID: 26967003 Review.
Cited by
-
Lu S, Jia Z, Meng X, Chen Y, Wang S, Fu C, Yang L, Zhou R, Wang B, Cao Y. Lu S, et al. Int J Mol Sci. 2022 Nov 16;23(22):14172. doi: 10.3390/ijms232214172. Int J Mol Sci. 2022. PMID: 36430648 Free PMC article.
-
Identification and Expression Analysis of UPS Gene Family in Potato.
Huang W, Lu Y, Ren B, Zeng F, Liu Y, Lu L, Li L. Huang W, et al. Genes (Basel). 2024 Jul 2;15(7):870. doi: 10.3390/genes15070870. Genes (Basel). 2024. PMID: 39062649 Free PMC article.
-
Govta N, Fatiukha A, Govta L, Pozniak C, Distelfeld A, Fahima T, Beckles DM, Krugman T. Govta N, et al. Theor Appl Genet. 2024 Jul 17;137(8):187. doi: 10.1007/s00122-024-04692-z. Theor Appl Genet. 2024. PMID: 39020219 Free PMC article.
-
Raihan MRH, Rahman M, Rastogi A, Fujita M, Hasanuzzaman M. Raihan MRH, et al. Antioxidants (Basel). 2023 Jul 27;12(8):1508. doi: 10.3390/antiox12081508. Antioxidants (Basel). 2023. PMID: 37627503 Free PMC article.
-
Rangani G, Rouse CE, Saski C, Noorai RE, Shankar V, Lawton-Rauh AL, Werle IS, Roma-Burgos N. Rangani G, et al. Genes (Basel). 2022 Mar 15;13(3):515. doi: 10.3390/genes13030515. Genes (Basel). 2022. PMID: 35328069 Free PMC article.
References
-
- Aoyama C, Santa T, Tsunoda M, Fukushima T, Kitada C, Imai K. 2004. A fully automated amino acid analyzer using NBD-F as a fluorescent derivatization reagent. Biomedical Chromatography 18, 630–636. - PubMed
-
- Atkins CA, Beevers L. 1990. Synthesis, transport and utilization of translocated solutes of nitrogen. In: Abrol YP, ed. Nitrogen in higher plants. Somerset, UK: Research Studies Press, 223–295.
-
- Atkins CA, Smith PMC. 2000. Ureide synthesis in legume nodules. In: Triplett EJ, ed. Prokaryotic nitrogen fixation: a model system for the analysis of a biological process. Wymondham, UK: Horizon Scientific Press, 559–587.
Publication types
MeSH terms
Substances
LinkOut - more resources
Full Text Sources