Mass Spectrometry-Based Protein Footprinting for Higher-Order Structure Analysis: Fundamentals and Applications - PubMed
- ️Wed Jan 01 2020
Review
Mass Spectrometry-Based Protein Footprinting for Higher-Order Structure Analysis: Fundamentals and Applications
Xiaoran Roger Liu et al. Chem Rev. 2020.
Abstract
Proteins adopt different higher-order structures (HOS) to enable their unique biological functions. Understanding the complexities of protein higher-order structures and dynamics requires integrated approaches, where mass spectrometry (MS) is now positioned to play a key role. One of those approaches is protein footprinting. Although the initial demonstration of footprinting was for the HOS determination of protein/nucleic acid binding, the concept was later adapted to MS-based protein HOS analysis, through which different covalent labeling approaches "mark" the solvent accessible surface area (SASA) of proteins to reflect protein HOS. Hydrogen-deuterium exchange (HDX), where deuterium in D2O replaces hydrogen of the backbone amides, is the most common example of footprinting. Its advantage is that the footprint reflects SASA and hydrogen bonding, whereas one drawback is the labeling is reversible. Another example of footprinting is slow irreversible labeling of functional groups on amino acid side chains by targeted reagents with high specificity, probing structural changes at selected sites. A third footprinting approach is by reactions with fast, irreversible labeling species that are highly reactive and footprint broadly several amino acid residue side chains on the time scale of submilliseconds. All of these covalent labeling approaches combine to constitute a problem-solving toolbox that enables mass spectrometry as a valuable tool for HOS elucidation. As there has been a growing need for MS-based protein footprinting in both academia and industry owing to its high throughput capability, prompt availability, and high spatial resolution, we present a summary of the history, descriptions, principles, mechanisms, and applications of these covalent labeling approaches. Moreover, their applications are highlighted according to the biological questions they can answer. This review is intended as a tutorial for MS-based protein HOS elucidation and as a reference for investigators seeking a MS-based tool to address structural questions in protein science.
Figures
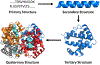
Four orders of protein structure exemplified by human deoxyhemoglobin (PDB ID 2HHB).
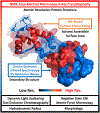
A summary of commonly used biophysical tools for characterizing protein HOS. Protein structure exemplified by calcium-free bovine calmodulin (PDB ID 1CFD)
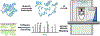
Schematic illustration of a bottom-up peptide mapping workflow, a necessary step prior to HDX. Green dots in the protein structure indicate hydrogen atoms in the peptide bonds.
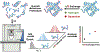
Schematic illustration of a bottom-up HDX workflow. Green and red dots in the protein structure indicate hydrogen and deuterium atoms in the peptide bonds, respectively.
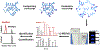
Schematic illustration of bottom-up protein HOS analysis through irreversible labeling approaches
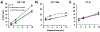
GEE labeling kinetics for selected IL-6R peptides in the ligand-free (gridded circle), adnectin 1-bound (triangle) and adnectin 2-bound state (diamond) state. (a) Region 135–148 shows decreased GEE incorporation upon adnectin 1/adnectin2 binding, whereas (b) region 274–284 shows increased GEE modification upon adnectin1 binding. (c) Representative peptide region without differentiable GEE modification extent between bound and unbound as a control. Dashed trend curves in (a), (b), and (c) are generated by linear or 2nd-degree polynomial fitting. Figure adopted with permission from Ref. . Copyright 2017 American Chemistry Society.
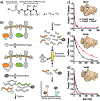
Overview of Cys footprinting with an ICAT reagent. (a) Structure of bromoacetamide ICAT reagent. Dots represent 12C for light reagent and 13C for heavy reagent. (b) Workflow for alkylation rate determination by ICAT isotope pairs. (c) – (e) Representative alkylation time courses of CheW Cys variants footprinted in the presence (red) or absence (black) of CheA*. Figure adopted with permission from Ref. . Copyright 2011 Elsevier.
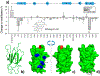
Covalent labeling results of β2m binding with rifamycin SV. (a) Changes in labeling modification percentages with rifamycin SV bound to the Cu(II)–protein complex. BD is 2,3-butanedione. Error bars represent standard deviations from triplicate measurements. Asterisks above the bars represent the residues that undergo a significant change in modification level at 95% confidence as determined by a two-sample unpaired Student’s t test. The arrows at the top of the graph indicate the locations and directions of the seven β strands in β2m. (b) Ribbon structure of β2m, showing the seven β strands labeled A through G. (c) β2m surface structure illustrating the sites undergoing significant changes in covalent labeling induced by rifamycin SV. Sites that increase in labeling are colored red, whereas those that decrease in labeling are colored blue. (d) Protein–ligand docking results for verification. Figure adopted with permission from Ref. . Copyright 2017 American Chemistry Society.
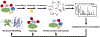
Workflow of bottom-up cross-linking mass spectrometry
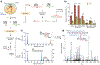
Schematic illustration of in vivo Cys footprinting workflow. (a) Scheme of MS analysis. The redox status of cysteines was quantified by differential isotope footprinting with NEM before and after a reduction step (right). Intact disulfide bonds were detected under nonreducing conditions (bottom). (b) Intracellular cysteine status was determined by quantitative MS before (red) and after DTT reduction (green). Bar graphs show means and errors of multiple peptides from three independent experiments **P < 0.001; ***P < 0.0001; #P > 0.05 by two-tailed Student’s t test. (c) Extracted-ion chromatograms showing a Cys51-Cys132 disulfide-linked peptide (top), which separates into two individual peptides (bottom) after reduction of the disulfide. A reference peptide is used as the standard (Std) for peak comparison. (d) MS/MS spectrum of the Cys51-Cys132 linked peptide (from c). Adopted with permission from Ref. . Copyright 2017 Springer Nature.
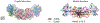
Representative model of the tetrameric tryptophan synthase and its corresponding crystal structure. Inter-residue proximities (XL-MS) and residue solvent accessibilities (covalent labeling MS, CL-MS) are highlighted. Figure adopted with permission from Ref. . Copyright 2017 American Chemistry Society.
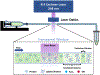
Schematic illustration of FPOP setup
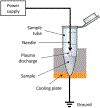
Schematic illustration of PLIMB setup. Adapted with permission from ref.. Copyright 2017 Springer Nature.
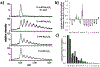
(a) The ESI-quadrupole time-of-flight mass spectra of the 15th charge state of β-lactoglobulin submitted to different labeling conditions as shown in the figure. (b) The relative difference in fraction modified between persulfate and the H2O2 approaches for CaM, aMb, bradykinin, and angiotensin II residues, are averaged per amino acid type. The error bars denote the average pairwise-comparison standard error. (c) The maximum fraction modified among all the same amino acid residues is plotted. Black bars denote H2O2 FPOP. Reprinted with permission from Ref. Copyright 2010 American Chemical Society.
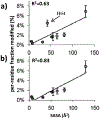
The modification yields of apomyoglobin His residues, where (a) all histidines were included and (b) His64 was omitted, are plotted against their calculated SASA, shown with least-squares straight-line fits. The SASA values were calculated by using a 1.4 Å probe. Reprinted with permission from Ref. Copyright 2010 American Chemical Society.
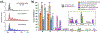
(a) The quadrupole time-of-flight (QTOF) mass spectra of the + 20 charge state of apo-myoglobin (aMb) under different labeling conditions as given in the figure. (b) Comparison of the residue-level fraction modified (in percentage) of a ‘Peptide Cocktail’ by CO3−• and ●OH. Different colors represent different peptides, and the corresponding sequences are shown. Solid bars denote the ●OH oxidation extent, and patterned bars denote the CO3−• oxidation. The data were corrected with respect to a negative control. Error bars are the standard deviations of three independent runs. The inset is an enlarged portion of the figure. For bradykinin, only F undergoes oxidation with CO3−•, whereas F and P are both oxidized by ●OH but not distinguishable because the chromatograms of the two modified peptides overlap. Reprinted with permission from Ref. Copyright 2019 Elsevier.
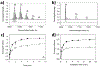
(a) Deconvoluted mass spectra of myoglobin after footprinting with carbenes. (b) Deconvoluted mass spectra of labeled and unlabeled holo-CaM. (c) Ca2+-binding induces conformational change on calmodulin where apo-calmodulin (closed circles) and holo-calmodulin (open circles) were labeled with 100 mM photoleucine in phosphate buffer and monitored as function of time. (d) Free holo-calmodulin (filled circles) is referenced to M13-bound holo-calmodulin (open circles). Reprinted with permission from Ref. . Copyright 2011 American Chemical Society.
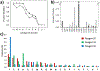
(a) Fractional distributions of carbene label derived from reagent 1 as determined by ETD (z-ions, filled circles) and HCD (y-ions, open circles) for peptide LTDEEVDEMIR (117–127) in CaM. (b) Residue-level reagent incorporation for select residues, based on ETD fragmentation of MKDTDSEEEIR (77–87), VFDKDGNGYISAAELR (92–107) and LTDEEVDEMIR (117–127) with Reagent 1 (gray bars) and Reagent 2 (black bars). Error bars are ± 1 standard deviation. Reprinted with permission from Ref. Copyright 2012 American Chemical Society. (c) Average frequency of carbene insertion at each residue generated from the photolysis of reagent 2, 3, and 4 in the presence of protein digests (777 peptides). Site of the label insertion was located with MS/MS with a Fusion Lumos with EThcD fragmentation and analyzed with Mass Spec Studio software. Reprinted with permission from Ref.. Copyright 2017 Springer Nature.
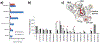
(a) Extent of labelling of a range of proteins with reagent 1 (100 mM, 16 s irradiation), and reagent 5 (10 mM, 4 s irradiation (*1 s in the case of CaM)). (b) Fractional modification by reagent 5 of USP5 peptides in the presence (black bars) and absence (white bars) of di-ubiquitin. Error bars are ± standard deviations (n = 3) and significant differences (Student’s t-test, p < 0.05) are highlighted with a red dot. (c) Model of USP5 (based on PDB 3IHP) showing the locations of the five peptides (red) that are masked from labelling by di-ubiquitin binding and their relative locations to the ZnFUBP and catalytic domains. Reprinted with permission from Ref. Copyright 2016 Springer Nature.
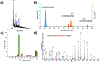
(a) Deconvoluted mass spectrum of CF3-modified aMb. (b) EIC of unmodified and CF3-modified peptide 32–45 of aMb. (c) Comparison of the extent of modification at the residue level for hMb and aMb. (d) A key mass shift of +68 Da is consistent with an unusual Gly labeling, here CF3 modification on Gly-276 of peptide 267–83, a glycine with large SASA. Reprinted with permission from Ref. Copyright 2017 John Wiley & Sons, Inc.
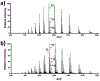
(a) Full ESI mass spectra of iodinated hMb. (b) Full ESI mass spectrum of iodinated aMb. The unmodified, mono-, di-, and tri-iodinated species of the 15th charge state are indicated by the number of stars. Reprinted with permission from Ref. . Copyright 2012 Springer Nature.
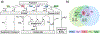
Summary of radical-based footprinting reagents of (a) proposed pathways and (b) residue specificity.
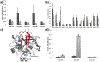
(a) Extent of modification of thrombin alone (darker bars) and antibody-bound thrombin (lighter bars) for the five peptides that span the 130-171 region. (b) Extent of modification of thrombin alone (darker bars) compared to antibody-bound thrombin (lighter bars) at the residue level. (c) Structural model of thrombin (PDB file 2AFQ) with the proposed epitope colored in red and the loop regions colored in blue. The individual residues that show increased modification for antibody-bound thrombin are specified. (d) Extent of modification of peptides from thrombin alone (darker bars) and antibody-bound thrombin (lighter bars) show increased solvent accessibility in the antibody-bound form. Reproduced with permission from ref . Copyright 2011 American Chemical Society.
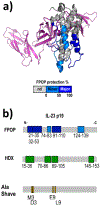
(a) Epitope regions determined by FPOP mapped on the crystal structure of IL-23 p19 domain. Color code: no significant difference (gray), minor epitope region (cyan), and major epitope region (blue). The p40 subunit is colored in purple. (b) Epitope regions determined by FPOP, HDX, and Ala shave energetics are mapped on the linear sequence of the IL-23 p19 domain. M3, D3, E9, L9 stands for M35A, D36A, E93A, L97A, respectively. Adapted with permission from ref . Copyright 2011 American Chemical Society.
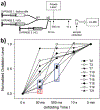
(a) Schematic illustration of the three-syringe, continuous-flow, rapid mixing setup for oxidative ●OH labeling. (b) Normalized oxidation levels of tryptic peptides plotted as a function of unfolding time t. Blue boxes highlight three peptides that retain considerable protection at 50 and 500 ms whereas the peptide highlighted by a red box is protected at 50 ms but not at 500 ms. Adapted with permission from ref . Copyright 2009 American Chemical Society.
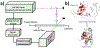
(a) Schematic illustration of the flow system intersected by two laser beams at a transparent window, as part of a temperature-jump apparatus. Adapted with permission from ref . Copyright 2009 American Chemical Society. (b) Proposed intermediate for barstar early folding. Residues colored in red, pink and gray represent residues that are closely associated with hydrophobic core, weakly associated with hydrophobic core and not involved in early folding intermediate, respectively. Adapted with permission from ref . Copyright 2009 American Chemical Society.
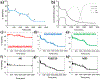
FPOP and kinetic modeling results for time-dependent aggregation of 42-residue Aβ (Aβ1-42) at (a, b) global, peptide and residue levels. (a) Global level results for Aβ1-42 aggregation. Solid curve is from a fitting model based on two autocatalytic reactions. (b) Concentrations for representative species (M-monomer, D-paranuclei, D*-protofibrils, D**-fibrils) as a function of incubation time based on kinetic simulation. (c, d, e) Peptide-level results for Lys-N digested Aβ1-42, N-terminal region 1-15, middle region 16-27 and C-terminal region 28-42, respectively. (f, g, h) Residue level results for three representative residues, H6, F19/F20 and M35, respectively. Points in each plot represent experimental data (10 μM, pH 7.4, no agitation) and error bars are standard deviations from three independent trials. Solid and dashed lines in (c – h) are model fits independent of or constrained by the global rates, respectively. Adapted with permission from ref . Copyright 2009 American Chemical Society.
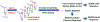
Schematic illustration LITPOMS workflow. Reprinted with permission from ref. . Copyright 2019 Springer.
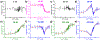
LITPOMS response of Ca2+ - CaM titration at peptide level, where modification fractions were plotted as a function of calcium:calmodulin concentration ratio. Four different classes of behaviors are shown in black (a and c), magenta (b), blue (d, f and h) and olive (e and g). Red solid lines in (b), (d), (e), (f), (g) and (h) are from fitting by a model used in PLIMSTEX. Reprinted with permission from ref . Copyright 2019 American Chemical Society.
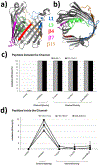
In vivo labeling of OmpF porin by hydroxyl radicals. 3D structure of OmpF (PDB ID 1OPF) from (a) side view and (b) top view. Structural components of loop 1, 3 and β-sheet 4, 7, 15 are highlighted in blue, green, red, purple and orange, respectively. Oxidation efficiency for (c) loops and (d) β-sheets under different experimental conditions are plotted. Error bars are standard deviations from duplicates. (c) and (d) are reprinted with permission from ref . Copyright 2009 American Society for Biochemistry and Molecular Biology.
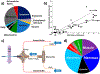
(a) Pie chart of the subcellular compartment location (African green monkey kidney cell) of oxidized proteins that were identified by LC-MS/MS. Proteins that are present in multiple compartments are represented multiple times. Reprinted with permission from ref . Copyright 2015 American Chemical Society. (b) Correlation of residue-level FPOP modifications with SASA in the open (circles and solid line) and tight (diamond and dashed line) states of actin. Reprinted with permission from ref. Copyright 2015 American Chemical Society. (c) Schematic illustration of an improved flow system for in vivo FPOP labeling. Blue arrows indicate flow and colored lines represent tubing. Reprinted with permission from ref. Copyright 2016 American Chemical Society. (d) Pie chart of the oxidatively modified proteins within different body systems of c. elegans. Reprinted with permission from ref. Copyright 2019 American Chemical Society.
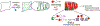
Schematic illustration of APEX labeling workflow. Reprinted with permission from ref. . Copyright 2013 American Association for the Advancement of Science.
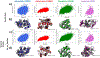
(a) Rosetta score versus RMSD (with respect to the native structure) plots for 20000 models generated from Rosetta ab initio for each of four proteins. Top scoring model is highlighted by a star in each plot. (b) Top scoring models from the Rosetta score versus RMSD distributions in (a) (color) superimposed on the respective native model (gray). PDB ID for these native models are depicted at the top of the figure. (c) Rosetta score + hrf_ms_labeling versus RMSD (with respect to the native structure) plots for each of the four proteins after rescoring with the new score term. The top-scoring model is highlighted by a star in each plot. (d) Top scoring models from the Rosetta score + hrf_ms_labeling rescoring distributions in (c) (color) superimposed on the respective native model (gray). Reprinted with permission from ref . Copyright 2018 American Chemical Society.
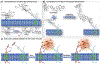
Schematic illustration of POSE workflow. (a) The incorporation of ManNAz into cell surface glycoproteins as SiaNAz. (b) The bio-orthogonal reaction of the DBCO-FeBABE probe with the SiaNAc group conducted via click-chemistry. (c) The oxidation of proteins in the sialic acid environment by ●OH generated by Fenton Chemistry. Reprinted with permission from ref . Copyright 2019 Royal Society of Chemistry.
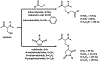
Cys labeling pathways with different labeling reagents
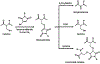
Cys labeling pathways with bromomaleimides
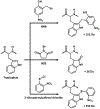
Trp labeling pathways with various reagents
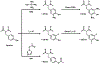
Tyr labeling pathways with several reagents
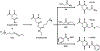
Asp and Glu labeling pathways with EDC-activated labeling reagents as demonstrated by Asp
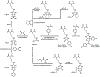
Arg labeling pathways with several reagents
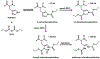
His labeling pathways with DEPC
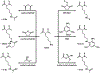
Lys labeling pathways with several reagents
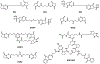
Common NHS-ester cross-linkers
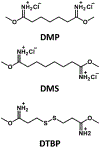
Common imidoester cross-linkers
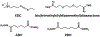
Common carbodiimide and dihydrazide cross-linkers
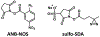
Chemical structure of common photoreactive cross-linkers, ANB-NOS and sulfo-SDA
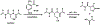
Free Cys footprinting by NTCB-mediated cleavage
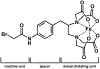
Structure of Fe-BABE

General scheme that produces reactive oxygen species during metal-catalyzed oxidation reactions.
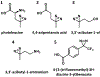
Diazirine-based footprinting reagents
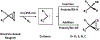
Proposed carbene reaction pathway
Similar articles
-
Li KS, Shi L, Gross ML. Li KS, et al. Acc Chem Res. 2018 Mar 20;51(3):736-744. doi: 10.1021/acs.accounts.7b00593. Epub 2018 Feb 16. Acc Chem Res. 2018. PMID: 29450991 Free PMC article. Review.
-
Liu XR, Rempel DL, Gross ML. Liu XR, et al. Nat Protoc. 2020 Dec;15(12):3942-3970. doi: 10.1038/s41596-020-0396-3. Epub 2020 Nov 9. Nat Protoc. 2020. PMID: 33169002 Free PMC article.
-
Protein Footprinting Comes of Age: Mass Spectrometry for Biophysical Structure Assessment.
Wang L, Chance MR. Wang L, et al. Mol Cell Proteomics. 2017 May;16(5):706-716. doi: 10.1074/mcp.O116.064386. Epub 2017 Mar 8. Mol Cell Proteomics. 2017. PMID: 28275051 Free PMC article. Review.
-
Garcia NK, Deperalta G, Wecksler AT. Garcia NK, et al. Protein Pept Lett. 2019;26(1):35-43. doi: 10.2174/0929866526666181128141953. Protein Pept Lett. 2019. PMID: 30484396 Review.
-
Rand KD, Zehl M, Jørgensen TJ. Rand KD, et al. Acc Chem Res. 2014 Oct 21;47(10):3018-27. doi: 10.1021/ar500194w. Epub 2014 Aug 29. Acc Chem Res. 2014. PMID: 25171396 Review.
Cited by
-
Xie Y, Sheng Y, Li Q, Ju S, Reyes J, Lebrilla CB. Xie Y, et al. Chem Sci. 2020 Aug 17;11(35):9501-9512. doi: 10.1039/d0sc04199h. Chem Sci. 2020. PMID: 34094216 Free PMC article.
-
Applications of MALDI-MS/MS-Based Proteomics in Biomedical Research.
Darie-Ion L, Whitham D, Jayathirtha M, Rai Y, Neagu AN, Darie CC, Petre BA. Darie-Ion L, et al. Molecules. 2022 Sep 21;27(19):6196. doi: 10.3390/molecules27196196. Molecules. 2022. PMID: 36234736 Free PMC article. Review.
-
Shah A, Batabyal D, Qiu D, Cui W, Harrahy J, Ivanov AR. Shah A, et al. J Pharm Anal. 2024 Aug;14(8):100966. doi: 10.1016/j.jpha.2024.100966. Epub 2024 Mar 16. J Pharm Anal. 2024. PMID: 39263356 Free PMC article.
-
Computational insight into stability-enhanced systems of anthocyanin with protein/peptide.
Xing C, Chen P, Zhang L. Xing C, et al. Food Chem (Oxf). 2023 Feb 21;6:100168. doi: 10.1016/j.fochms.2023.100168. eCollection 2023 Jul 30. Food Chem (Oxf). 2023. PMID: 36923156 Free PMC article.
-
Cheng Z, Misra SK, Shami A, Sharp JS. Cheng Z, et al. Anal Chem. 2022 Dec 27;94(51):18017-18024. doi: 10.1021/acs.analchem.2c04365. Epub 2022 Dec 13. Anal Chem. 2022. PMID: 36512753 Free PMC article.
References
-
- Lodish H; Berk A; Kaiser CA; Krieger M; Scott MP; Bretscher A; Ploegh H; Matsudaira P, Molecular cell biology. Macmillan: 2008.
-
- Fermi G; Perutz MF; Shaanan B; Fourme R, The crystal structure of human deoxyhaemoglobin at 1.74 Å resolution. Journal of Molecular Biology 1984, 175 (2), 159–174. - PubMed
-
- Mathews CKVH, K. E.; Appling DR; Anthony-Cahill SJ, Biochemistry. Pearson: 2013.
-
- FRUTON JS, Early Theories of Protein Structure. Annals of the New York Academy of Sciences 1979, 325 (1), 1–20. - PubMed
Publication types
MeSH terms
Substances
LinkOut - more resources
Full Text Sources
Research Materials