Stigmasterol Causes Ovarian Cancer Cell Apoptosis by Inducing Endoplasmic Reticulum and Mitochondrial Dysfunction - PubMed
- ️Wed Jan 01 2020
Stigmasterol Causes Ovarian Cancer Cell Apoptosis by Inducing Endoplasmic Reticulum and Mitochondrial Dysfunction
Hyocheol Bae et al. Pharmaceutics. 2020.
Abstract
Background: Phytosterols have physiological effects and are used as medicines or food supplements. Stigmasterol has shown anticancer effects against various cancers such as hepatoma, cholangiocarcinoma, gall bladder carcinoma, endometrial adenocarcinoma and skin, gastric, breast, prostate, and cervical cancer. However, there are no reports on stigmasterol's effects on ovarian cancer.
Methods: We investigated the effects of stigmasterol on proapoptotic signals, mitochondrial function, reactive oxygen species production, and the cytosolic and mitochondrial calcium levels in human ovarian cancer cells, to understand the mechanisms underlying the effects of stigmasterol on ovarian cancer cells. We also conducted migration assay to confirm whether that stigmasterol inhibits ovarian cancer cell migration.
Results: Stigmasterol inhibited development of human ovarian cancer cells. However, it induced cell apoptosis, ROS production, and calcium overload in ES2 and OV90 cells. In addition, stigmasterol stimulated cell death by activating the ER-mitochondrial axis. We confirmed that stigmasterol suppressed cell migration and angiogenesis genes in human ovarian cancer cells.
Conclusions: Our findings suggest that stigmasterol can be used as a new treatment for ovarian cancer.
Keywords: apoptosis; endoplasmic reticulum; migration; mitochondria; ovarian cancer; stigmasterol.
Conflict of interest statement
The authors declare no conflicts of interest.
Figures
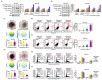
Stigmasterol affects ovarian cancer cell apoptosis and tumor formation in ES2 and OV90 cells. (A,B) Western blot bands showed the expression of proapoptotic signaling molecules in both cell types following stigmasterol treatments (0, 5, 10, and 20 μg/mL). Alpha-tubulin (TUBA) was used as a control. (C,D) Spheroid formation of ovarian cancer cells was compared between vehicle-treated cells and stigmasterol-treated cells. (E,F) Annexin V and propidium iodide (PI) staining were performed to determine cell death in ES2 and OV90 cells. The quadrant of the dot blot represents the state of apoptosis in ES2 and OV90 cells. The comparative graph represents changes in late apoptosis due to stigmasterol treatment (0, 5, 10, and 20 μg/mL) compared to the vehicle-treated control (100%) in ES2 and OV90 cells. (G,H) Histogram presents cell cycle progression in stigmasterol-treated (0, 5, 10, and 20 μg/mL) ovarian cancer cells. Comparative graph represents the % of cells in the subG1, G0/G1, S, and G2/M phases in stigmasterol-treated (0, 5, 10, and 20 μg/mL) ovarian cancer cells.
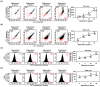
Alteration of mitochondrial membrane potential and ROS generation by stigmasterol in both cells. (A,B) JC-1 dye was used to investigate the alteration of mitochondrial membrane potential by stigmasterol (0, 5, 10, and 20 μg/mL). Quadrant of the dot blot represented the state of mitochondrial membrane potential in ES2 and OV90 cells. Comparative graph represented the loss of the mitochondrial membrane potential compared to the control group (100%) in ES2 and OV90 cells. (C,D) DCF fluorescence intensity was observed to investigate the change by stigmasterol treatment (0, 5, 10, and 20 μg/mL). The histogram represented the state of ROS production in ES2 (Figure 2C, left) and OV90 (Figure 2D, left) cells. Comparative graph represented ROS production compared to vehicle-treated control (100%) in ES2 (Figure 2C, right) and OV90 (Figure 2D, right) cells. DCF: dichlorofluorescein.
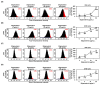
Calcium concentrations in the cytosol and mitochondria in stigmasterol-treated ovarian cancer cells. (A,B) Fluo-4 dye was used to determine the intracellular calcium levels. The histogram represents the change of cytosolic calcium levels following stigmasterol treatments (0, 5, 10, and 20 μg/mL) in ES2 (Figure 3A, left) and OV90 (Figure 3B, left) cells. The comparative graph represents changes in intracellular calcium levels following stigmasterol treatments compared to the vehicle-treated controls (100%) in ES2 (Figure 3A, right) and OV90 (Figure 3B, right) cells. (C,D) Rhod-2 fluorescence was used to determine the mitochondrial calcium levels in stigmasterol-treated ovarian cancer cells. The histogram represents changes in mitochondrial calcium levels following stigmasterol treatments (0, 5, 10, and 20 μg/mL) in ES2 (Figure 3C, left) and OV90 (Figure 3D, left) cells. The comparative graph represents changes in mitochondrial calcium levels following stigmasterol treatments compared to the vehicle-treated control (100%) in ES2 (Figure 3C, right) and OV90 (Figure 3D, right) cells.
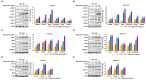
Increased expression of endoplasmic reticulum (ER) stress sensor proteins and ER-mitochondrial axis proteins due to stigmasterol treatment in ovarian cancer cells. (A,B) Immunoblots representing the expression of unfolded protein response (UPR) proteins following stigmasterol treatments (0, 5, 10, and 20 μg/mL). TUBA was used as a control and is shown at the bottom of the figure. (C,D) Immunoblots representing the expression of ER-mitochondrial axis proteins following stigmasterol treatments (0, 5, 10, and 20 μg/mL). TUBA was used as a control and is shown at the bottom of the figure. (E,F) Immunoblots representing the expression of autophagy proteins following stigmasterol treatment. TUBA was used as a control and is shown at the bottom of the figure.
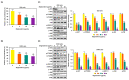
Restriction of cell growth and cell growth-related signals in ovarian cancer cells following stigmasterol treatments. (A,B) Cell proliferation data shows the cell viability (%) following the treatment of ovarian cancer cells with stigmasterol. The comparative graph represents the relative proliferation of each cell type following treatments with stigmasterol, compared to vehicle-treated control cells (100%). (C,D) Western blots present the changes of PI3K and MAPK expression following the treatments of ovarian cancer cells with stigmasterol (0, 5, 10, and 20 μg/mL).
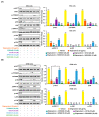
Cotreatment of stigmasterol and inhibitors in ovarian cancer cells. (A,B) Western blots showed changes of PI3K and MAPK by cotreatment with stigmasterol or each inhibitor in ovarian cancer cells. Comparative graph represented the change of phosphorylation compared to the vehicle-treated control (100%) in ES2 and OV90 cells.
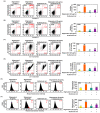
Calcium regulation and cell apoptosis by stigmasterol in ovarian cancer. (A,B) Annexin V and PI were observed to investigate the cell death of ES2 and OV90 cells. Quadrant of the dot blot represented the state of cell apoptosis in ES2 and OV90 cells. Comparative graph represented the change of late apoptosis by stigmasterol or a combination with ruthenium red compared to the control (100%) in ES2 and OV90 cells. (C,D) JC-1 dye was used to investigate the alteration of the mitochondrial membrane potential by stigmasterol or a combination with ruthenium red. Quadrant of the dot blot represented the state of the mitochondrial membrane potential. Comparative graph represented the loss of the mitochondrial membrane potential compared to the control group (100%). (E,F) Rhod-2 fluorescence was observed to investigate the mitochondrial calcium levels in stigmasterol or a combination with ruthenium red. The histogram represented a change of the mitochondrial calcium. Comparative graph represented the change of the mitochondrial calcium levels.
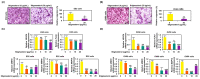
Reduced cell migration by stigmasterol in ovarian cancer cells. (A,B) Cell migration was observed using Transwell inserts. Migrated cells through the membrane were measured on images of five nonoverlapping locations. (C,D) Gene expression of migration-related genes were investigated by quantitative real-time (RT)-PCR. All experiments were conducted in triplicate. Scale bar represents 100 μm.
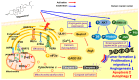
Schematic illustration of the mechanisms downstream of stigmasterol in human ovarian cancer cells.
Similar articles
-
Elucidation of the Chemopreventive Role of Stigmasterol Against Jab1 in Gall Bladder Carcinoma.
Pandey P, Bajpai P, Siddiqui MH, Sayyed U, Tiwari R, Shekh R, Mishra K, Kapoor VK. Pandey P, et al. Endocr Metab Immune Disord Drug Targets. 2019;19(6):826-837. doi: 10.2174/1871530319666190206124120. Endocr Metab Immune Disord Drug Targets. 2019. PMID: 30727937
-
Xue J, Li R, Zhao X, Ma C, Lv X, Liu L, Liu P. Xue J, et al. Chem Biol Interact. 2018 Mar 1;283:59-74. doi: 10.1016/j.cbi.2018.02.003. Epub 2018 Feb 5. Chem Biol Interact. 2018. PMID: 29421517
-
Disruption of Endoplasmic Reticulum and ROS Production in Human Ovarian Cancer by Campesterol.
Bae H, Park S, Yang C, Song G, Lim W. Bae H, et al. Antioxidants (Basel). 2021 Mar 3;10(3):379. doi: 10.3390/antiox10030379. Antioxidants (Basel). 2021. PMID: 33802602 Free PMC article.
-
Health Benefits and Pharmacological Properties of Stigmasterol.
Bakrim S, Benkhaira N, Bourais I, Benali T, Lee LH, El Omari N, Sheikh RA, Goh KW, Ming LC, Bouyahya A. Bakrim S, et al. Antioxidants (Basel). 2022 Sep 27;11(10):1912. doi: 10.3390/antiox11101912. Antioxidants (Basel). 2022. PMID: 36290632 Free PMC article. Review.
-
Advances in Stigmasterol on its anti-tumor effect and mechanism of action.
Zhang X, Wang J, Zhu L, Wang X, Meng F, Xia L, Zhang H. Zhang X, et al. Front Oncol. 2022 Dec 12;12:1101289. doi: 10.3389/fonc.2022.1101289. eCollection 2022. Front Oncol. 2022. PMID: 36578938 Free PMC article. Review.
Cited by
-
Zhang Q, Zhang L, Liu K, Shang H, Ruan J, Yu Z, Meng S, Liang F, Wang T, Zhang H, Peng W, Wang Y, Chen J, Xiao T, Wang B. Zhang Q, et al. Evid Based Complement Alternat Med. 2022 Feb 8;2022:1638740. doi: 10.1155/2022/1638740. eCollection 2022. Evid Based Complement Alternat Med. 2022. PMID: 35178098 Free PMC article.
-
Ramalingam AP, Mohanavel W, Premnath A, Muthurajan R, Prasad PVV, Perumal R. Ramalingam AP, et al. Antioxidants (Basel). 2021 Sep 23;10(10):1511. doi: 10.3390/antiox10101511. Antioxidants (Basel). 2021. PMID: 34679645 Free PMC article.
-
Guo M, Zeng J, Wang Z, Shen Y. Guo M, et al. Heliyon. 2024 Apr 12;10(8):e29557. doi: 10.1016/j.heliyon.2024.e29557. eCollection 2024 Apr 30. Heliyon. 2024. PMID: 38644901 Free PMC article. Review.
-
Phytochemicals in Gynecological Cancer Prevention.
Woźniak M, Krajewski R, Makuch S, Agrawal S. Woźniak M, et al. Int J Mol Sci. 2021 Jan 26;22(3):1219. doi: 10.3390/ijms22031219. Int J Mol Sci. 2021. PMID: 33530651 Free PMC article. Review.
-
Sun J, Liu J, Liu D, Wu X. Sun J, et al. Biomed Res Int. 2020 Oct 8;2020:8965459. doi: 10.1155/2020/8965459. eCollection 2020. Biomed Res Int. 2020. PMID: 33150184 Free PMC article.
References
LinkOut - more resources
Full Text Sources