Electrode Materials in Modern Organic Electrochemistry - PubMed
- ️Wed Jan 01 2020
Review
. 2020 Oct 19;59(43):18866-18884.
doi: 10.1002/anie.202005745. Epub 2020 Aug 24.
Affiliations
- PMID: 32633073
- PMCID: PMC7589451
- DOI: 10.1002/anie.202005745
Review
Electrode Materials in Modern Organic Electrochemistry
David M Heard et al. Angew Chem Int Ed Engl. 2020.
Abstract
The choice of electrode material is critical for achieving optimal yields and selectivity in synthetic organic electrochemistry. The material imparts significant influence on the kinetics and thermodynamics of electron transfer, and frequently defines the success or failure of a transformation. Electrode processes are complex and so the choice of a material is often empirical and the underlying mechanisms and rationale for success are unknown. In this review, we aim to highlight recent instances of electrode choice where rationale is offered, which should aid future reaction development.
Keywords: electrocatalysis; electrochemistry; electrode; materials; organic synthesis.
© 2020 The Authors. Published by Wiley-VCH GmbH.
Conflict of interest statement
The authors declare no conflict of interest.
Figures
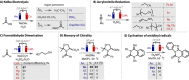
Examples of the effect of electrode materials on classic (A–D) and contemporary (E) reactions. Gr.=graphite, GC=glassy carbon; RVC=reticulated vitreous carbon.
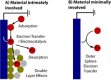
The two limiting cases for electron‐transfer.

HER and OER overpotentials taken from Table 1 (averaged where relevant) for various electrode materials.
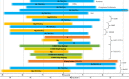
Solvent windows various electrode material and electrolyte combinations.33, 123, 124, 125, 126, 127, 128, 129 († current density cut‐off at j=±0.1 mA cm−2.).
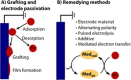
Electrode passivation.
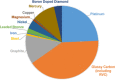
Occurrence of electrode materials used (cathode or anode) in a survey of 915 synthetic electrochemical protocols published between 2000–2017.
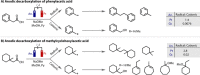
Product distributions arising from anodic decarboxylation on either platinum or graphite electrodes.
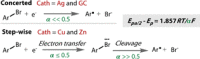
Mechanistic elucidation of reductive debromination on different cathode materials.
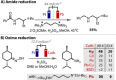
A,B) Cathode material with a high overpotential for proton reduction is necessary for attaining good selectivity for substrate reduction.
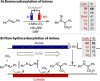
A) Increased adsorption of imine on Ag cathode leading to decomposition and dimerization avoided. B) Materials with high overpotential for CO2 reduction required for attaining highest product yields.
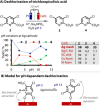
A) Unique electrocatalytic behaviour of Ag exploited for dechlorination of 2. with trend at different pHs. B) Proposed pH dependent adsorption mode.
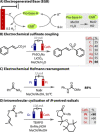
A) Generation of a base on the cathode. B–D) A low overpotential for proton reduction on the counter electrode renders a milder generation of a base, which improves product yields.
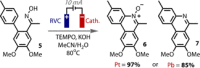
Low overpotential for proton reduction on Pt counter electrode gives 6, but high overpotential on Pb means 6 is preferentially reduced to give 7.
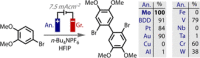
Surface modification through in situ generation of active MoV coating on anode.
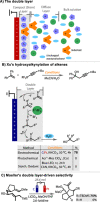
A) Cartoon of the interfacial double layer formed at a cathode; B) double layer‐controlled selectivity of nucleophile attack; C) exclusion of MeOH from double layer promotes cyclisation.
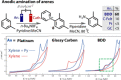
Anodic amination of arenes by Waldvogel showing unique performance of BDD anode. Cyclic voltammograms of m‐xylene (red) and m‐xylene with pyridine (blue), on platinum, glassy carbon and BDD. Green dashed box highlights the unaffected oxidation feature of xylene upon addition of pyridine on BDD anode.
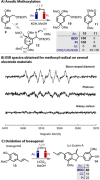
A) Two possible mechanisms for the oxidative methoxylation of 9 (a) and (b) to give 10. Aldehyde 11 is formed from (c); B) ESR spectra reveal methoxyl radicals, leading to mechanism (b); C) formation of methoxyl radicals are more efficient on BDD anode in oxidation of isoeugenol.
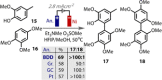
BDD gives best yields for phenol/arene coupling reaction, which produces reactive radical intermediates.
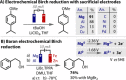
A) Mg as sacrificial electrode leads to most efficient reactivity; B) Mg or Al as sacrificial electrode. Mg2+ proposed not to play significant role in mechanism.
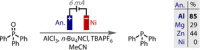
Phosphine oxide deoxygenation is aided by sacrificial Al anode for in situ Lewis acid generation.
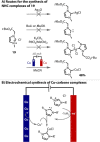
A) NHC complexes generated from an electrochemical process; B) the mechanism for their formation.
Similar articles
-
Exner KS, Over H. Exner KS, et al. Acc Chem Res. 2017 May 16;50(5):1240-1247. doi: 10.1021/acs.accounts.7b00077. Epub 2017 May 2. Acc Chem Res. 2017. PMID: 28463492
-
Bipolar Electrochemistry: A Powerful Tool for Electrifying Functional Material Synthesis.
Shida N, Zhou Y, Inagi S. Shida N, et al. Acc Chem Res. 2019 Sep 17;52(9):2598-2608. doi: 10.1021/acs.accounts.9b00337. Epub 2019 Aug 22. Acc Chem Res. 2019. PMID: 31436076
-
Patil B, Kobayashi Y, Fujikawa S, Okajima T, Mao L, Ohsaka T. Patil B, et al. Bioelectrochemistry. 2014 Feb;95:15-22. doi: 10.1016/j.bioelechem.2013.10.005. Epub 2013 Oct 19. Bioelectrochemistry. 2014. PMID: 24189123
-
Advances in Electrocatalysis for Energy Conversion and Synthesis of Organic Molecules.
Holade Y, Servat K, Tingry S, Napporn TW, Remita H, Cornu D, Kokoh KB. Holade Y, et al. Chemphyschem. 2017 Oct 6;18(19):2573-2605. doi: 10.1002/cphc.201700447. Epub 2017 Sep 12. Chemphyschem. 2017. PMID: 28732139 Review.
-
A Decade of Electrochemical Dehydrogenative C,C-Coupling of Aryls.
Röckl JL, Pollok D, Franke R, Waldvogel SR. Röckl JL, et al. Acc Chem Res. 2020 Jan 21;53(1):45-61. doi: 10.1021/acs.accounts.9b00511. Epub 2019 Dec 18. Acc Chem Res. 2020. PMID: 31850730 Review.
Cited by
-
Prenzel T, Schwarz N, Hammes J, Krähe F, Pschierer S, Winter J, Gálvez-Vázquez MJ, Schollmeyer D, Waldvogel SR. Prenzel T, et al. Org Process Res Dev. 2024 Sep 24;28(10):3922-3928. doi: 10.1021/acs.oprd.4c00337. eCollection 2024 Oct 18. Org Process Res Dev. 2024. PMID: 39444427 Free PMC article.
-
Practical electrochemical hydrogenation of nitriles at the nickel foam cathode.
Narobe R, Perner MN, Gálvez-Vázquez MJ, Kuhwald C, Klein M, Broekmann P, Rösler S, Cezanne B, Waldvogel SR. Narobe R, et al. Green Chem. 2024 Sep 5;26(20):10567-10574. doi: 10.1039/d4gc03446e. eCollection 2024 Oct 14. Green Chem. 2024. PMID: 39309016 Free PMC article.
-
Abdeljaoued A, Ruiz BL, Tecle YE, Langner M, Bonakdar N, Bleyer G, Stenner P, Vogel N. Abdeljaoued A, et al. Nat Commun. 2024 Jun 27;15(1):5437. doi: 10.1038/s41467-024-48142-2. Nat Commun. 2024. PMID: 38937451 Free PMC article.
-
Electrocatalysis as an enabling technology for organic synthesis.
Novaes LFT , Liu J , Shen Y , Lu L , Meinhardt JM , Lin S . Novaes LFT , et al. Chem Soc Rev. 2021 Jul 21;50(14):7941-8002. doi: 10.1039/d1cs00223f. Epub 2021 Jun 1. Chem Soc Rev. 2021. PMID: 34060564 Free PMC article.
-
Zhu Z, Daboczi M, Chen M, Xuan Y, Liu X, Eslava S. Zhu Z, et al. Nat Commun. 2024 Mar 30;15(1):2791. doi: 10.1038/s41467-024-47100-2. Nat Commun. 2024. PMID: 38555394 Free PMC article.
References
-
- Kärkäs M. D., Chem. Soc. Rev. 2018, 47, 5786–5865. - PubMed
Publication types
LinkOut - more resources
Full Text Sources
Other Literature Sources