Long-Running Comparison of Feed-Water Scaling in Membrane Distillation - PubMed
- ️Wed Jan 01 2020
Long-Running Comparison of Feed-Water Scaling in Membrane Distillation
Mohammad Rezaei et al. Membranes (Basel). 2020.
Abstract
Membrane distillation (MD) has shown promise for concentrating a wide variety of brines, but the knowledge is limited on how different brines impact salt scaling, flux decline, and subsequent wetting. Furthermore, past studies have lacked critical details and analysis to enable a physical understanding, including the length of experiments, the inclusion of salt kinetics, impact of antiscalants, and variability between feed-water types. To address this gap, we examined the system performance, water recovery, scale formation, and saturation index of a lab-scale vacuum membrane distillation (VMD) in long-running test runs approaching 200 h. The tests provided a comparison of a variety of relevant feed solutions, including a synthetic seawater reverse osmosis brine with a salinity of 8.0 g/L, tap water, and NaCl, and included an antiscalant. Saturation modeling indicated that calcite and aragonite were the main foulants contributing to permeate flux reduction. The longer operation times than typical studies revealed several insights. First, scaling could reduce permeate flux dramatically, seen here as 49% for the synthetic brine, when reaching a high recovery ratio of 91%. Second, salt crystallization on the membrane surface could have a long-delayed but subsequently significant impact, as the permeate flux experienced a precipitous decline only after 72 h of continuous operation. Several scaling-resistant impacts were observed as well. Although use of an antiscalant did not reduce the decrease in flux, it extended membrane operational time before surface foulants caused membrane wetting. Additionally, numerous calcium, magnesium, and carbonate salts, as well as silica, reached very high saturation indices (>1). Despite this, scaling without wetting was often observed, and scaling was consistently reversible and easily washed. Under heavy scaling conditions, many areas lacked deposits, which enabled continued operation; existing MD performance models lack this effect by assuming uniform layers. This work implies that longer times are needed for MD fouling experiments, and provides further scaling-resistant evidence for MD.
Keywords: antiscalant; long-term performance tests; reverse osmosis brine; scaling; vacuum membrane distillation; wetting.
Conflict of interest statement
The authors declare no conflict of interest.
Figures
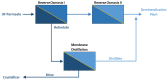
Water desalination by an RO + VMD integrated process. The schematic diagram shows the hybrid reverse-osmosis membrane-distillation process for maximizing the system recovery rate and minimizing the environmental impact of brine waste when a VMD unit is added downstream of the RO unit for further recovery.
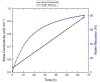
Brine conductivity and water recovery as functions of time for the system with tap water as feed.
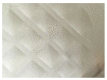
Membrane surface after the NaCl experiment.
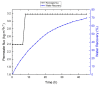
Permeate flux and water recovery as functions of time when using an aqueous NaCl solution as feed.
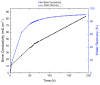
Brine conductivity and water recovery as functions of time for the synthetic brine feed system without antiscalant.
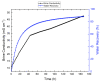
Brine conductivity and water recovery as functions of time for the synthetic brine feed system with antiscalant.
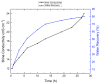
Effect of forced (i.e., high-pressure) wetting on system performance.
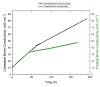
Effect of scaling on system performance.
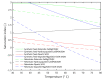
Aqueous speciation equilibrium of synthetic RO feed and retentate. Solid lines indicate the saturation indices of secondary precipitated minerals in the synthetic RO feed, while dashed lines indicate the corresponding saturation indices for the retentate. Modeled using PHREEQC software. At low temperatures, silica minerals typically form colloids rather than precipitates. Dolomite requires temperatures above 100 °C for precipitation activation.
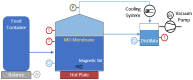
Vacuum membrane distillation (VMD) process schematic. The setup consisted of a feed container, a stirred membrane cell, a distillate collection vessel, a feed container, a magnetic stirrer, an external condenser, a balance, and a vacuum pump.
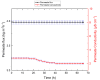
Linz tap water VMD test showing permeate flux and permeate electrical conductivity against time. The system exhibited no reduction in permeate flux during the experiment.
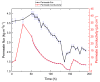
Permeate flux and permeate electrical conductivity as functions of time for the synthetic feed system without antiscalant. The permeate flux decreased, which indicates fouling, but permeate electrical conductivity remained at low levels, which indicates the absence of wetting. Feed temperature, permeate temperature, and gauge pressure were kept constant at 75 °C, 21 °C, and −30 mbar during the experiments, respectively.
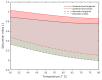
Aqueous speciation equilibrium of synthetic RO brine feed without antiscalant and its corresponding retentate. Solid lines indicate the saturation indices of the main mineral precipitates in synthetic RO brine feed without antiscalant, while dashed lines indicate the corresponding saturation indices for the retentate. The shaded area indicates the drop in saturation indices caused by precipitation on the membrane surface. Modeled using PHREEQC software.
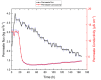
Permeate flux and permeate electrical conductivity as functions of time for the synthetic brine feed system with antiscalant. The antiscalant led to higher initial permeate flux compared to the system without antiscalant. Permeate electrical conductivity remained relatively low.
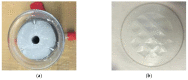
After the experiment with synthetic RO brine: (a) Membrane module; (b) Membrane surface. Salt crystals deposits are visible, which can easily be removed by washing with water.
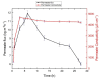
Effect of forced (i.e., high-pressure) wetting on system performance. Operation of the process above liquid entry pressure (LEP) led to rapid passage of liquid feed to the permeate side of the membrane, which drastically increased permeate electrical conductivity.
Similar articles
-
Vacuum membrane distillation of seawater reverse osmosis brines.
Mericq JP, Laborie S, Cabassud C. Mericq JP, et al. Water Res. 2010 Oct;44(18):5260-73. doi: 10.1016/j.watres.2010.06.052. Epub 2010 Jul 7. Water Res. 2010. PMID: 20659753
-
Son HS, Soukane S, Lee J, Kim Y, Kim YD, Ghaffour N. Son HS, et al. J Environ Manage. 2021 Sep 1;293:112836. doi: 10.1016/j.jenvman.2021.112836. Epub 2021 May 27. J Environ Manage. 2021. PMID: 34052611
-
Yao Y, Ge X, Yin Y, Minjarez R, Tong T. Yao Y, et al. Water Res. 2023 Nov 1;246:120701. doi: 10.1016/j.watres.2023.120701. Epub 2023 Oct 5. Water Res. 2023. PMID: 37837901
-
Fouling and wetting in the membrane distillation driven wastewater reclamation process - A review.
Choudhury MR, Anwar N, Jassby D, Rahaman MS. Choudhury MR, et al. Adv Colloid Interface Sci. 2019 Jul;269:370-399. doi: 10.1016/j.cis.2019.04.008. Epub 2019 Apr 27. Adv Colloid Interface Sci. 2019. PMID: 31129338 Review.
-
Milne NA, O'Reilly T, Sanciolo P, Ostarcevic E, Beighton M, Taylor K, Mullett M, Tarquin AJ, Gray SR. Milne NA, et al. Water Res. 2014 Nov 15;65:107-33. doi: 10.1016/j.watres.2014.07.010. Epub 2014 Jul 12. Water Res. 2014. PMID: 25105586 Review.
Cited by
-
Chandrasekaran AS, Fix AJ, Warsinger DM. Chandrasekaran AS, et al. Membranes (Basel). 2022 Mar 22;12(4):348. doi: 10.3390/membranes12040348. Membranes (Basel). 2022. PMID: 35448318 Free PMC article.
References
-
- Ahmed M., Arakel A., Hoey D., Thumarukudy M.R., Goosen M.F.A., Al-Haddabi M., Al-Belushi A. Feasibility of salt production from inland RO desalination plant reject brine: A case study. Desalination. 2003;158:109–117. doi: 10.1016/S0011-9164(03)00441-7. - DOI
-
- Singh D., Sirkar K.K. Desalination of brine and produced water by direct contact membrane distillation at high temperatures and pressures. J. Memb. Sci. 2012;389:380–388. doi: 10.1016/j.memsci.2011.11.003. - DOI
-
- Mericq J.-P., Laborie S., Cabassud C. Vacuum membrane distillation for an integrated seawater desalination process. Desalin. Water Treat. 2009;9:287–296. doi: 10.5004/dwt.2009.862. - DOI
LinkOut - more resources
Full Text Sources
Miscellaneous