Nanoelectromechanical Sensors Based on Suspended 2D Materials - PubMed
- ️Wed Jan 01 2020
Review
. 2020 Jul 20:2020:8748602.
doi: 10.34133/2020/8748602. eCollection 2020.
Stefan Wagner 2 , Kangho Lee 3 , Xuge Fan 4 , Gerard J Verbiest 5 , Sebastian Wittmann 6 , Sebastian Lukas 1 , Robin J Dolleman 7 , Frank Niklaus 4 , Herre S J van der Zant 8 , Georg S Duesberg 3 , Peter G Steeneken 5 8
Affiliations
- PMID: 32766550
- PMCID: PMC7388062
- DOI: 10.34133/2020/8748602
Review
Nanoelectromechanical Sensors Based on Suspended 2D Materials
Max C Lemme et al. Research (Wash D C). 2020.
Abstract
The unique properties and atomic thickness of two-dimensional (2D) materials enable smaller and better nanoelectromechanical sensors with novel functionalities. During the last decade, many studies have successfully shown the feasibility of using suspended membranes of 2D materials in pressure sensors, microphones, accelerometers, and mass and gas sensors. In this review, we explain the different sensing concepts and give an overview of the relevant material properties, fabrication routes, and device operation principles. Finally, we discuss sensor readout and integration methods and provide comparisons against the state of the art to show both the challenges and promises of 2D material-based nanoelectromechanical sensing.
Copyright © 2020 Max C. Lemme et al.
Conflict of interest statement
The authors declare that there are no conflicts of interest regarding the publication of this article.
Figures
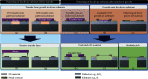
2D NEMS device fabrication methods. (a–e) Create a 2D material layer on the device substrate, where for (a) and (b) the device substrate is prepatterned and for (c–e) the substrate is patterned afterwards. (f)–(j) show post 2D material layer fabrication steps to create suspended membranes.
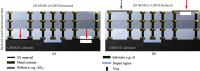
CMOS integration of 2D NEMS sensors in backend. (a) NEMS sensor devices integrated in the backend with interconnect layers stacked on top and frontend. (b) Integration of the 2D material in the frontend on top of the interconnect layers. The silicon IC substrate (dark grey) with transistors (blue) and interconnect metals (gray/yellow) is shown. Red arrows indicate the location of the black suspended graphene.
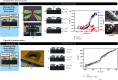
Piezoresistive NEMS pressure sensor. (a) Fabrication method of the suspended membrane (according to Figure 1). (b, c) Example device image [6]. (d–f) Working principle: pressure difference causes tension which alters the membrane resistance by the piezoresistive effect. (g) Graphene piezoresistive pressure sensor measurement [25]. Capacitive pressure sensor: (h) fabrication of the suspended membrane. (i) Device schematic [182]. (j–l) Working principle: a pressure difference causes the membrane to deflect and alter the capacitance between the graphene and the bottom electrode. (m) Device measurement [182].
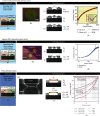
Tension-induced pressure sensor: (a) fabrication method of the suspended membrane (according to Figure 1) and (b) example device [21]. (c–e) Working principle: the gas pressure difference across the membrane causes a membrane deflection and tension change that is measured via the resonance frequency. (f) Graphene tension-induced pressure sensor measurement [21]. Squeeze-film pressure sensor: (g) fabrication of the suspended membrane and (h) example device [175]. (i, j) Working principle: the stiffness and compressibility of the gas under the membrane increases the stiffness of the membrane that is measured via the mechanical resonance frequency. (k) Example measurement of a graphene-based squeeze-film pressure sensor [175]. Graphene Pirani pressure sensor: (l) fabrication of the suspended membrane; (m) example device of a Pirani pressure sensor [132]. (n, o) Working principle: the temperature, and temperature-dependent resistance, of the suspended, Joule-heated graphene beam depends on the pressure-dependent gas cooling rate. (p) Example measurement of a Pirani pressure sensor based on graphene [132].
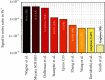
SNR comparison of piezoresistive (PR), capacitive (CAP), and squeeze-film (SQF) MEMS pressure sensors. Included are Wagner et al. [6], Murata SCB10H [184], Dolleman et al. [175], Stampfer et al. [188], Epcos C35 [200], Zhang et al. [172], Wang et al. [185], Davidovikj et al. [182], and Smith et al. [25].
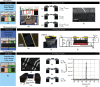
Microphone: (a) fabrication method of the suspended membrane (according to Figure 1); (b, f) images of an example device [146]. (c–e) Working principle: the sound pressure-dependent deflection of the membrane is detected via its capacitance with respect to the backplate. Ultrasound detector: (a) fabrication of the suspended membrane; (h) example device [181] and (i, j) working principle: the graphene membrane is moved by the ultrasound-induced motion of its supports, and its motion is detected using transconductance readout. Accelerometer: (k) fabrication of the suspended membrane; (l, m) example device [72], (n–p) working principle: the acceleration-induced forces on the suspended mass cause tension in the graphene that is detected using the piezoresistive effect. (q) The output signal of an accelerometer [72].
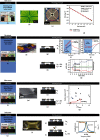
Hall sensor: (a) fabrication method of the suspended membrane (according to Figure 1) and (b, c) example device [146] and readout of an example device [146]. Gas sensor: (e) fabrication of the suspended membrane and ribbon and (f) example device [244]. (g, h) Working principle: gas molecules adhere to the (functionalized) 2D material and alter its resistance via electronic or chemical interactions. (i, j) Readout of an example device [248] and (k) typical sensor response plot of MoSe2 sensors depending on electron-donating/withdrawing gas [110]. Mass sensor: (l) fabrication of the suspended membrane, (m) example device, and (n, o) working principle: by measuring the resonance frequency, the mass change of the membrane is derived. (p) Extracted mass and tension of the membrane during multiple loading cycles [83]. Bolometer: (q) fabrication of the suspended membrane and ribbon and (r) example device [266]. (s, t) Working principle: when radiation heats the membrane, this alters its tension and causes a shift in mechanical resonance frequency. (u) Readout of an example device with a graphene membrane [266].
Similar articles
-
Nanomaterials Based Micro/Nanoelectromechanical System (MEMS and NEMS) Devices.
Torkashvand Z, Shayeganfar F, Ramazani A. Torkashvand Z, et al. Micromachines (Basel). 2024 Jan 24;15(2):175. doi: 10.3390/mi15020175. Micromachines (Basel). 2024. PMID: 38398905 Free PMC article. Review.
-
Liu Q, He C, Ding J, Zhang W, Fan X. Liu Q, et al. ACS Appl Mater Interfaces. 2024 Oct 30;16(43):59066-59076. doi: 10.1021/acsami.4c11941. Epub 2024 Oct 19. ACS Appl Mater Interfaces. 2024. PMID: 39425792
-
Kajale SN, Yadav S, Cai Y, Joy B, Sarkar D. Kajale SN, et al. iScience. 2021 Nov 25;24(12):103513. doi: 10.1016/j.isci.2021.103513. eCollection 2021 Dec 17. iScience. 2021. PMID: 34934930 Free PMC article. Review.
-
Recent Development of Gas Sensing Platforms Based on 2D Atomic Crystals.
Cao J, Chen Q, Wang X, Zhang Q, Yu HD, Huang X, Huang W. Cao J, et al. Research (Wash D C). 2021 Apr 21;2021:9863038. doi: 10.34133/2021/9863038. eCollection 2021. Research (Wash D C). 2021. PMID: 33982003 Free PMC article. Review.
-
A review on two-dimensional materials for chemiresistive- and FET-type gas sensors.
Zhang J, Liu L, Yang Y, Huang Q, Li D, Zeng D. Zhang J, et al. Phys Chem Chem Phys. 2021 Jul 28;23(29):15420-15439. doi: 10.1039/d1cp01890f. Phys Chem Chem Phys. 2021. PMID: 34263272
Cited by
-
Haub M, Bogner M, Guenther T, Zimmermann A, Sandmaier H. Haub M, et al. Sensors (Basel). 2021 May 30;21(11):3795. doi: 10.3390/s21113795. Sensors (Basel). 2021. PMID: 34070885 Free PMC article.
-
Stacking Polymorphism in PtSe2 Drastically Affects Its Electromechanical Properties.
Kempt R, Lukas S, Hartwig O, Prechtl M, Kuc A, Brumme T, Li S, Neumaier D, Lemme MC, Duesberg GS, Heine T. Kempt R, et al. Adv Sci (Weinh). 2022 Aug;9(22):e2201272. doi: 10.1002/advs.202201272. Epub 2022 Jun 2. Adv Sci (Weinh). 2022. PMID: 35652199 Free PMC article.
-
Cho C, Zhang Z, Kim JM, Ma PJ, Haque MF, Snapp P, Nam S. Cho C, et al. Nano Lett. 2023 Oct 25;23(20):9340-9346. doi: 10.1021/acs.nanolett.3c02550. Epub 2023 Oct 5. Nano Lett. 2023. PMID: 37796972 Free PMC article.
-
High-Yield Large-Scale Suspended Graphene Membranes over Closed Cavities for Sensor Applications.
Lukas S, Esteki A, Rademacher N, Jangra V, Gross M, Wang Z, Ngo HD, Bäuscher M, Mackowiak P, Höppner K, Wehenkel DJ, van Rijn R, Lemme MC. Lukas S, et al. ACS Nano. 2024 Sep 17;18(37):25614-25624. doi: 10.1021/acsnano.4c06827. Epub 2024 Sep 8. ACS Nano. 2024. PMID: 39244663 Free PMC article.
-
Monolayer MXene Nanoelectromechanical Piezo-Resonators with 0.2 Zeptogram Mass Resolution.
Tan D, Cao X, Huang J, Peng Y, Zeng L, Guo Q, Sun N, Bi S, Ji R, Jiang C. Tan D, et al. Adv Sci (Weinh). 2022 Aug;9(22):e2201443. doi: 10.1002/advs.202201443. Epub 2022 May 26. Adv Sci (Weinh). 2022. PMID: 35619285 Free PMC article.
References
-
- Hill E. W., Vijayaragahvan A., Novoselov K. Graphene sensors. IEEE Sensors Journal. 2011;11(12):3161–3170. doi: 10.1109/JSEN.2011.2167608. - DOI
Publication types
LinkOut - more resources
Full Text Sources