From Synaptic Dysfunction to Neuroprotective Strategies in Genetic Parkinson's Disease: Lessons From LRRK2 - PubMed
- ️Wed Jan 01 2020
Review
From Synaptic Dysfunction to Neuroprotective Strategies in Genetic Parkinson's Disease: Lessons From LRRK2
Andrea Mancini et al. Front Cell Neurosci. 2020.
Abstract
The pathogenesis of Parkinson's disease (PD) is thought to rely on a complex interaction between the patient's genetic background and a variety of largely unknown environmental factors. In this scenario, the investigation of the genetic bases underlying familial PD could unveil key molecular pathways to be targeted by new disease-modifying therapies, still currently unavailable. Mutations in the leucine-rich repeat kinase 2 (LRRK2) gene are responsible for the majority of inherited familial PD cases and can also be found in sporadic PD, but the pathophysiological functions of LRRK2 have not yet been fully elucidated. Here, we will review the evidence obtained in transgenic LRRK2 experimental models, characterized by altered striatal synaptic transmission, mitochondrial dysfunction, and α-synuclein aggregation. Interestingly, the processes triggered by mutant LRRK2 might represent early pathological phenomena in the pathogenesis of PD, anticipating the typical neurodegenerative features characterizing the late phases of the disease. A comprehensive view of LRRK2 neuronal pathophysiology will support the possible clinical application of pharmacological compounds targeting this protein, with potential therapeutic implications for patients suffering from both familial and sporadic PD.
Keywords: LRRK2; Parkinson’s disease; mitochondrial dysfunction; neuroprotection; synaptic dysfunction; α-synuclein.
Copyright © 2020 Mancini, Mazzocchetti, Sciaccaluga, Megaro, Bellingacci, Beccano-Kelly, Di Filippo, Tozzi and Calabresi.
Figures
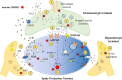
Striatal synaptic effects of leucine-rich repeatkinase 2 (LRRK2). LRRK2 is thought to influence striatal synaptogenesis interacting with cytoskeleton and microtubules ①. The modulation of presynaptic voltage-gated Ca2+ channels (CaV2.1; ②), as well as the regulation of synaptic vesicle exocytosis, endocytosis, and recycling ③, could influence the release of glutamate (Glu) from the corticostriatal excitatory terminal ④. Moreover, the postsynaptic expression of glutamatergic AMPAR could be influenced by LRRK2 activity ⑤. LRRK2 could alter striatal DAergic transmission ⑥ inducing midbrain neuronal loss or DAergic terminals abnormalities, including abnormal DAT activity ⑦ and pathological α-synuclein aggregation ⑧. The postsynaptic expression and function of DA receptors can be influenced by mutant LRRK2, including altered D2R-dependent postsynaptic synthesis of endocannabinoids (eCBs; ⑨) and D1R expression/internalization ⑩. Finally, the dysfunction of PKA and DARPP32 pathways ⑪ and the impairment of intracellular AMPAR exocytosis ⑫ may result in altered synaptic long-term changes. AC, adenylate cyclase; CaMKII, Ca2+-calmodulin dependent protein kinase II; cAMP, 3′,5′-cyclic adenosine monophosphate; CB1R, cannabinoid receptor type 1; ER, endoplasmic reticulum; IP3, inositol trisphosphate; PLC, phospholipase C.
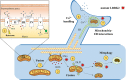
Suggested mitochondrial effects of mutant LRRK2. Enhanced susceptibility to rotenone, a mitochondrial chain complex I inhibitor, was found in genetic LRRK2 experimental models ①. This increased susceptibility to mitochondrial dysfunction could impair ATP formation and enhance reactive oxygen species (ROS) production ② after the exposure to endogenous or exogenous mitochondrial stressors. Moreover, LRRK2 is hypothesized to alter mitochondrial fusion ③ and fission ④ process, by interacting with mitochondrial docking proteins, such as Miro, or dynamin-like protein 1 (DLP1). Abnormally active LRRK2 could also impair the removal of damaged mitochondria through lysosomal-dependent mitophagy ⑤ and alter mitochondrial Ca2+ buffering activity ⑥ at mitochondria–ER interactions ⑦. On the upper left, simplified representation of the mitochondrial electron transport chain (including mitochondrial complexes I, II, III, IV, and V; coenzyme Q; and cytochrome c).
Similar articles
-
Andersen MA, Christensen KV, Badolo L, Smith GP, Jeggo R, Jensen PH, Andersen KJ, Sotty F. Andersen MA, et al. Neurobiol Dis. 2018 Aug;116:13-27. doi: 10.1016/j.nbd.2018.04.011. Epub 2018 Apr 20. Neurobiol Dis. 2018. PMID: 29680709
-
Chen J, Chen Y, Pu J. Chen J, et al. Eur Neurol. 2018;79(5-6):256-265. doi: 10.1159/000488938. Epub 2018 Apr 27. Eur Neurol. 2018. PMID: 29705795 Review.
-
Verma M, Callio J, Otero PA, Sekler I, Wills ZP, Chu CT. Verma M, et al. J Neurosci. 2017 Nov 15;37(46):11151-11165. doi: 10.1523/JNEUROSCI.3791-16.2017. Epub 2017 Oct 16. J Neurosci. 2017. PMID: 29038245 Free PMC article.
-
[Clinical molecular genetics for PARK8 (LRRK2)].
Tomiyama H, Hatano T, Hattori N. Tomiyama H, et al. Brain Nerve. 2007 Aug;59(8):839-50. Brain Nerve. 2007. PMID: 17713120 Review. Japanese.
-
Palomo-Garo C, Gómez-Gálvez Y, García C, Fernández-Ruiz J. Palomo-Garo C, et al. Pharmacol Res. 2016 Aug;110:181-192. doi: 10.1016/j.phrs.2016.04.004. Epub 2016 Apr 6. Pharmacol Res. 2016. PMID: 27063942
Cited by
-
Molecular Mechanisms Underlying Synaptic and Axon Degeneration in Parkinson's Disease.
Gcwensa NZ, Russell DL, Cowell RM, Volpicelli-Daley LA. Gcwensa NZ, et al. Front Cell Neurosci. 2021 Mar 2;15:626128. doi: 10.3389/fncel.2021.626128. eCollection 2021. Front Cell Neurosci. 2021. PMID: 33737866 Free PMC article.
-
Update on CSF Biomarkers in Parkinson's Disease.
Kwon EH, Tennagels S, Gold R, Gerwert K, Beyer L, Tönges L. Kwon EH, et al. Biomolecules. 2022 Feb 18;12(2):329. doi: 10.3390/biom12020329. Biomolecules. 2022. PMID: 35204829 Free PMC article. Review.
-
Setzu MD, Mocci I, Fabbri D, Carta P, Muroni P, Diana A, Dettori MA, Casu MA. Setzu MD, et al. Biomolecules. 2024 Feb 24;14(3):273. doi: 10.3390/biom14030273. Biomolecules. 2024. PMID: 38540694 Free PMC article.
-
Zhang Q, Cheng X, Wu W, Yang S, You H, Ye Z, Liu N, Chen X, Pan X. Zhang Q, et al. J Mol Neurosci. 2022 Mar;72(3):527-543. doi: 10.1007/s12031-021-01896-6. Epub 2021 Aug 19. J Mol Neurosci. 2022. PMID: 34409578
-
Future Prospects in Parkinson's Disease Diagnosis and Treatment.
Öksüz N, Öztürk Ş, Doğu O. Öksüz N, et al. Noro Psikiyatr Ars. 2022 Dec 16;59(Suppl 1):S36-S41. doi: 10.29399/npa.28169. eCollection 2022. Noro Psikiyatr Ars. 2022. PMID: 36578989 Free PMC article. Review.
References
Publication types
Grants and funding
LinkOut - more resources
Full Text Sources