De novo design of picomolar SARS-CoV-2 miniprotein inhibitors - PubMed
- ️Wed Jan 01 2020
. 2020 Oct 23;370(6515):426-431.
doi: 10.1126/science.abd9909. Epub 2020 Sep 9.
Inna Goreshnik 1 2 , Brian Coventry 1 2 3 , James Brett Case 4 , Lauren Miller 1 2 , Lisa Kozodoy 1 2 , Rita E Chen 4 5 , Lauren Carter 1 2 , Alexandra C Walls 1 , Young-Jun Park 1 , Eva-Maria Strauch 6 , Lance Stewart 1 2 , Michael S Diamond 4 7 , David Veesler 1 , David Baker 8 2 9
Affiliations
- PMID: 32907861
- PMCID: PMC7857403
- DOI: 10.1126/science.abd9909
De novo design of picomolar SARS-CoV-2 miniprotein inhibitors
Longxing Cao et al. Science. 2020.
Abstract
Targeting the interaction between the severe acute respiratory syndrome coronavirus 2 (SARS-CoV-2) spike protein and the human angiotensin-converting enzyme 2 (ACE2) receptor is a promising therapeutic strategy. We designed inhibitors using two de novo design approaches. Computer-generated scaffolds were either built around an ACE2 helix that interacts with the spike receptor binding domain (RBD) or docked against the RBD to identify new binding modes, and their amino acid sequences were designed to optimize target binding, folding, and stability. Ten designs bound the RBD, with affinities ranging from 100 picomolar to 10 nanomolar, and blocked SARS-CoV-2 infection of Vero E6 cells with median inhibitory concentration (IC50) values between 24 picomolar and 35 nanomolar. The most potent, with new binding modes, are 56- and 64-residue proteins (IC50 ~ 0.16 nanograms per milliliter). Cryo-electron microscopy structures of these minibinders in complex with the SARS-CoV-2 spike ectodomain trimer with all three RBDs bound are nearly identical to the computational models. These hyperstable minibinders provide starting points for SARS-CoV-2 therapeutics.
Copyright © 2020 The Authors, some rights reserved; exclusive licensee American Association for the Advancement of Science. No claim to original U.S. Government Works.
Figures
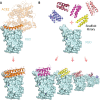
(A) Design of helical proteins incorporating ACE2 helix. (B) Large-scale de novo design of small helical scaffolds (top) followed by RIF docking to identify shape and chemically complementary binding modes.
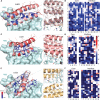
(A, C, and E) (Left) Designed binding proteins are colored by positional Shannon entropy from site saturation mutagenesis, with blue indicating positions of low entropy (conserved) and red those of high entropy (not conserved). (Right) Zoomed-in views of central regions of the design core and interface with the RBD. (B, D, and F) Heat maps representing RBD-binding enrichment values for single mutations in the design model core (left) and the designed interface (right). Substitutions that are heavily depleted are shown in blue, and beneficial mutations are shown in red. The depletion of most substitutions in both the binding site and the core suggest that the design models are largely correct, whereas the enriched substitutions suggest routes to improving affinity. Full SSM maps over all positions for AHB2 and all eight de novo designs are provided in figs. S6 and S7.
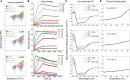
(A) ACE2 competes with the designs for binding to the RBD. Yeast cells displaying the indicated design were incubated with 200 pM RBD in the presence or absence of 1 μM ACE2, and RBD binding to cells (y axis) was monitored with flow cytometry. (B) Binding of purified miniproteins to the RBD monitored with BLI. For LCB1 and LCB3, dissociation constants (Kd) could not be accurately estimated because of a lack of instrument sensitivity and long equilibration times below 200 pM. (C) Circular dichroism spectra at different temperatures and (D) CD signal at 222-nm wavelength, as a function of temperature. The fully de novo designs LCB1 and LCB3 are more stable than the ACE2 scaffolded helix design AHB2.
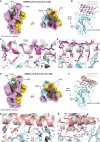
(A) Molecular surface representation of LCB1 bound to the SARS-CoV-2 spike ectodomain trimer viewed along two orthogonal axes (left, side view; right, top view) (B) Superimposition of the computational design model (silver) and refined cryo-EM structure (magenta) of LCB1 (using the map obtained through local refinement) bound to the RBD (cyan). (C and D) Zoomed-in views of computational model (silver) of LCB1/RBD complex overlaid on the cryo-EM structure (cyan for RBD and pink for LCB1), showing selected interacting side chains. (E) Molecular surface representation of LCB3 bound to the SARS-CoV-2 spike ectodomain trimer viewed from the side and top of the spike trimer. (F) Superimposition of the computational design model (silver) and refined cryo-EM structure (pink) of LCB3 (using the map obtained through local refinement) bound to the RBD (cyan). (G and H) Zoomed-in view of the interactions between LCB3 (pink) and the SARS-CoV-2 RBD (cyan), showing selected interacting side chains. In (A) and (E), each spike protomer is colored distinctly (cyan, pink, and yellow). For (B) and (F), the RBDs were superimposed to evaluate the binding pose deviations between designed models and refined structure of each minibinder.
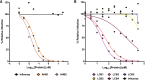
(A and B) Neutralization activity of (A) AHB1 and AHB2 or (B) LCB1-5 were measured with a focus reduction neutralization test. Indicated concentrations of minibinders were incubated with 100 FFU of authentic SARS-CoV-2 and subsequently transferred onto Vero E6 monolayers. AHB1, AHB2, LCB1, and LCB3 potently neutralize SARS-CoV-2, with median effective concentration (EC50) values <50 nM (AHB1 and AHB2) or <50 pM (LCB1 and LCB3). Data are representative of two independent experiments, each performed in technical duplicate.
Update of
-
De novo design of picomolar SARS-CoV-2 miniprotein inhibitors.
Cao L, Goreshnik I, Coventry B, Case JB, Miller L, Kozodoy L, Chen RE, Carter L, Walls L, Park YJ, Stewart L, Diamond M, Veesler D, Baker D. Cao L, et al. bioRxiv [Preprint]. 2020 Aug 3:2020.08.03.234914. doi: 10.1101/2020.08.03.234914. bioRxiv. 2020. PMID: 32793905 Free PMC article. Updated. Preprint.
Similar articles
-
An alpaca nanobody neutralizes SARS-CoV-2 by blocking receptor interaction.
Hanke L, Vidakovics Perez L, Sheward DJ, Das H, Schulte T, Moliner-Morro A, Corcoran M, Achour A, Karlsson Hedestam GB, Hällberg BM, Murrell B, McInerney GM. Hanke L, et al. Nat Commun. 2020 Sep 4;11(1):4420. doi: 10.1038/s41467-020-18174-5. Nat Commun. 2020. PMID: 32887876 Free PMC article.
-
De novo design of picomolar SARS-CoV-2 miniprotein inhibitors.
Cao L, Goreshnik I, Coventry B, Case JB, Miller L, Kozodoy L, Chen RE, Carter L, Walls L, Park YJ, Stewart L, Diamond M, Veesler D, Baker D. Cao L, et al. bioRxiv [Preprint]. 2020 Aug 3:2020.08.03.234914. doi: 10.1101/2020.08.03.234914. bioRxiv. 2020. PMID: 32793905 Free PMC article. Updated. Preprint.
-
Fantini J, Chahinian H, Yahi N. Fantini J, et al. Int J Antimicrob Agents. 2020 Aug;56(2):106020. doi: 10.1016/j.ijantimicag.2020.106020. Epub 2020 May 13. Int J Antimicrob Agents. 2020. PMID: 32405156 Free PMC article.
-
Progress in Studies on Structural and Remedial Aspects of Newly Born Coronavirus, SARS-CoV-2.
Gupta SP. Gupta SP. Curr Top Med Chem. 2020;20(26):2362-2378. doi: 10.2174/1568026620666200922112300. Curr Top Med Chem. 2020. PMID: 32962613 Review.
-
Inhibition of S-protein RBD and hACE2 Interaction for Control of SARSCoV- 2 Infection (COVID-19).
Nayak SK. Nayak SK. Mini Rev Med Chem. 2021;21(6):689-703. doi: 10.2174/1389557520666201117111259. Mini Rev Med Chem. 2021. PMID: 33208074 Review.
Cited by
-
Shehzadi K, Yu M, Liang J. Shehzadi K, et al. Int J Mol Sci. 2023 Dec 14;24(24):17473. doi: 10.3390/ijms242417473. Int J Mol Sci. 2023. PMID: 38139312 Free PMC article.
-
A pan-variant miniprotein inhibitor protects against SARS-CoV-2 variants.
Lee J, Case JB, Park YJ, Ravichandran R, Asarnow D, Tortorici MA, Brown JT, Sanapala S, Carter L, Baker D, Diamond MS, Veesler D. Lee J, et al. bioRxiv [Preprint]. 2024 Aug 10:2024.08.08.606885. doi: 10.1101/2024.08.08.606885. bioRxiv. 2024. PMID: 39149384 Free PMC article. Preprint.
-
Recombinant Protein Micelles to Block Transduction by SARS-CoV-2 Pseudovirus.
Chattaraj R, Kim CY, Lee D, Hammer DA. Chattaraj R, et al. ACS Nano. 2022 Oct 25;16(10):17466-17477. doi: 10.1021/acsnano.2c09015. Epub 2022 Oct 3. ACS Nano. 2022. PMID: 36191145 Free PMC article.
-
Stapled peptides as potential inhibitors of SARS-CoV-2 binding to the hACE2 receptor.
Tzotzos S. Tzotzos S. J Pept Sci. 2022 Sep;28(9):e3409. doi: 10.1002/psc.3409. Epub 2022 Feb 26. J Pept Sci. 2022. PMID: 35165970 Free PMC article. Review.
-
Protein design via deep learning.
Ding W, Nakai K, Gong H. Ding W, et al. Brief Bioinform. 2022 May 13;23(3):bbac102. doi: 10.1093/bib/bbac102. Brief Bioinform. 2022. PMID: 35348602 Free PMC article. Review.
References
-
- Hou Y. J., Okuda K., Edwards C. E., Martinez D. R., Asakura T., Dinnon K. H. 3rd, Kato T., Lee R. E., Yount B. L., Mascenik T. M., Chen G., Olivier K. N., Ghio A., Tse L. V., Leist S. R., Gralinski L. E., Schäfer A., Dang H., Gilmore R., Nakano S., Sun L., Fulcher M. L., Livraghi-Butrico A., Nicely N. I., Cameron M., Cameron C., Kelvin D. J., de Silva A., Margolis D. M., Markmann A., Bartelt L., Zumwalt R., Martinez F. J., Salvatore S. P., Borczuk A., Tata P. R., Sontake V., Kimple A., Jaspers I., O’Neal W. K., Randell S. H., Boucher R. C., Baric R. S., SARS-CoV-2 reverse genetics reveals a variable infection gradient in the respiratory tract. Cell 182, 429–446.e14 (2020). 10.1016/j.cell.2020.05.042 - DOI - PMC - PubMed
-
- Shi R., Shan C., Duan X., Chen Z., Liu P., Song J., Song T., Bi X., Han C., Wu L., Gao G., Hu X., Zhang Y., Tong Z., Huang W., Liu W. J., Wu G., Zhang B., Wang L., Qi J., Feng H., Wang F. S., Wang Q., Gao G. F., Yuan Z., Yan J., A human neutralizing antibody targets the receptor-binding site of SARS-CoV-2. Nature 584, 120–124 (2020). 10.1038/s41586-020-2381-y - DOI - PubMed
-
- D. Pinto, Y.-J. Park, M. Beltramello, A. C. Walls, M. A. Tortorici, S. Bianchi, S. Jaconi, K. Culap, F. Zatta, A. De Marco, A. Peter, B. Guarino, R. Spreafico, E. Cameroni, J. B. Case, R. E. Chen, C. Havenar-Daughton, G. Snell, A. Telenti, H. W. Virgin, A. Lanzavecchia, M. S. Diamond, K. Fink, D. Veesler, D. Corti, Structural and functional analysis of a potent sarbecovirus neutralizing antibody. bioRxiv 023903 [Preprint] 10 April 2020; 10.1101/2020.04.07.023903.10.1101/2020.04.07.023903 - DOI - DOI
Publication types
MeSH terms
Substances
Grants and funding
LinkOut - more resources
Full Text Sources
Other Literature Sources
Molecular Biology Databases
Miscellaneous