Relevance of the Warburg Effect in Tuberculosis for Host-Directed Therapy - PubMed
- ️Wed Jan 01 2020
Review
Relevance of the Warburg Effect in Tuberculosis for Host-Directed Therapy
Bridgette M Cumming et al. Front Cell Infect Microbiol. 2020.
Abstract
Tuberculosis (TB) was responsible for more deaths in 2019 than any other infectious agent. This epidemic is exacerbated by the ongoing development of multi-drug resistance and HIV co-infection. Recent studies have therefore focused on identifying host-directed therapies (HDTs) that can be used in combination with anti-mycobacterial drugs to shorten the duration of TB treatment and improve TB outcomes. In searching for effective HDTs for TB, studies have looked toward immunometabolism, the study of the role of metabolism in host immunity and, in particular, the Warburg effect. Across a variety of experimental paradigms ranging from in vitro systems to the clinic, studies on the role of the Warburg effect in TB have produced seemingly conflicting results and contradictory conclusions. To reconcile this literature, we take a historical approach to revisit the definition of the Warburg effect, re-examine the foundational papers on the Warburg effect in the cancer field and explore its application to immunometabolism. With a firm context established, we assess the literature investigating metabolism and immunometabolism in TB for sufficient evidence to support the role of the Warburg effect in TB immunity. The effects of the differences between animal models, species of origin of the macrophages, duration of infection and Mycobacterium tuberculosis strains used for these studies are highlighted. In addition, the shortcomings of using 2-deoxyglucose as an inhibitor of glycolysis are discussed. We conclude by proposing experimental criteria that are essential for future studies on the Warburg effect in TB to assist with the research for HDTs to combat TB.
Keywords: Warburg effect; host-directed therapy; immunometabolism; macrophage; tuberculosis.
Copyright © 2020 Cumming, Pacl and Steyn.
Figures
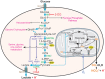
Roles of aerobic glycolysis. Aerobic glycolysis generates metabolic intermediates that are used in other biosynthetic pathways such as the pentose phosphate pathway, synthesis of hexosamines, glycerol-3-phosphate for fatty acid synthesis, amino acid synthesis, and one carbon metabolism. Aerobic glycolysis is also involved in maintaining redox balance (NAD+/NADH) in the cell. Inhibition of hexokinase (HK) in the first step of glycolysis by 2-DG will additionally inhibit these pathways and redox homeostasis. Thus, inhibitors reducing the glycolytic flux by acting on enzymes near the end of glycolytic pathway, such as OXA, on LDH, or LDHA knock-out mice will enable a more accurate assessment of the contribution of glycolysis to effector functions of the cell. This figure also demonstrates how both lactate from glycolysis and carbon dioxide generated from mitochondrial metabolism contribute to the acidification of the extracellular environment (ECAR). HK, hexokinase; 2-DG, 2-deoxyglucose; Glc-6-P, glucose-6-phosphate; Fru-6-P, fructose-6-phosphate; PFK, phosphofructokinase, Fru-1,6-biP, fructose-1, 6-bisphosphate; DHAP, dihydroxyacetone phosphate; GAPDH, glyceraldehyde phosphate dehydrogenase, 1,3-bis-PG, 1,3-bisphosphoglycerate; 3-PG, 2-PG, 3- or 2-phosphoglyerate; ENO, enolase; 2-PEP, 2-phosphoenolpyruvate; LDHA, lactate dehydrogenase A; OXA, sodium oxamate; OAA, oxaloacetate; α-KG, α-ketoglutarate.
Similar articles
-
[Development of antituberculous drugs: current status and future prospects].
Tomioka H, Namba K. Tomioka H, et al. Kekkaku. 2006 Dec;81(12):753-74. Kekkaku. 2006. PMID: 17240921 Review. Japanese.
-
[Frontier of mycobacterium research--host vs. mycobacterium].
Okada M, Shirakawa T. Okada M, et al. Kekkaku. 2005 Sep;80(9):613-29. Kekkaku. 2005. PMID: 16245793 Japanese.
-
Cahill C, Cox DJ, O'Connell F, Basdeo SA, Gogan KM, Ó'Maoldomhnaigh C, O'Sullivan J, Keane J, Phelan JJ. Cahill C, et al. Int J Mol Sci. 2021 Nov 10;22(22):12189. doi: 10.3390/ijms222212189. Int J Mol Sci. 2021. PMID: 34830070 Free PMC article.
-
Hawn TR, Shah JA, Kalman D. Hawn TR, et al. Immunol Rev. 2015 Mar;264(1):344-62. doi: 10.1111/imr.12255. Immunol Rev. 2015. PMID: 25703571 Free PMC article. Review.
-
Host factors subverted by Mycobacterium tuberculosis: Potential targets for host directed therapy.
Kalra R, Tiwari D, Dkhar HK, Bhagyaraj E, Kumar R, Bhardwaj A, Gupta P. Kalra R, et al. Int Rev Immunol. 2023;42(1):43-70. doi: 10.1080/08830185.2021.1990277. Epub 2021 Oct 22. Int Rev Immunol. 2023. PMID: 34678117 Review.
Cited by
-
Park JH, Shim D, Kim KES, Lee W, Shin SJ. Park JH, et al. Front Cell Infect Microbiol. 2021 Mar 16;11:635335. doi: 10.3389/fcimb.2021.635335. eCollection 2021. Front Cell Infect Microbiol. 2021. PMID: 33796480 Free PMC article. Review.
-
Lactate cross-talk in host-pathogen interactions.
Llibre A, Grudzinska FS, O'Shea MK, Duffy D, Thickett DR, Mauro C, Scott A. Llibre A, et al. Biochem J. 2021 Sep 17;478(17):3157-3178. doi: 10.1042/BCJ20210263. Biochem J. 2021. PMID: 34492096 Free PMC article. Review.
-
Ning Y, Cai Y, Dai Y, Li F, Mo S, Werz O, Chen X. Ning Y, et al. Infect Immun. 2021 Oct 15;89(11):e0030621. doi: 10.1128/IAI.00306-21. Epub 2021 Aug 9. Infect Immun. 2021. PMID: 34370506 Free PMC article.
-
Lactate Metabolism and Signaling in Tuberculosis and Cancer: A Comparative Review.
Kiran D, Basaraba RJ. Kiran D, et al. Front Cell Infect Microbiol. 2021 Feb 26;11:624607. doi: 10.3389/fcimb.2021.624607. eCollection 2021. Front Cell Infect Microbiol. 2021. PMID: 33718271 Free PMC article. Review.
-
Dias HF, Fu JF, Luck TG, Wolfe GE, Hostetter ER, Ng NC, Zheng H, Kühtreiber WM, Price JC, Catana C, Faustman DL. Dias HF, et al. Sci Rep. 2024 Jul 30;14(1):17611. doi: 10.1038/s41598-024-67905-x. Sci Rep. 2024. PMID: 39080423 Free PMC article.
References
-
- Baay-Guzman G. J., Duran-Padilla M. A., Rangel-Santiago J., Tirado-Rodriguez B., Antonio-Andres G., Barrios-Payan J., et al. . (2018). Dual role of hypoxia-inducible factor 1 alpha in experimental pulmonary tuberculosis: its implication as a new therapeutic target. Future Microbiol. 13, 785–798. 10.2217/fmb-2017-0168 - DOI - PubMed
-
- Bakker A. (1927). Einige übereinstimmungen im stoffwechsel der carcinomzellen und exsudatleukocyten. Klin. Wochenschr. 6, 252–254. 10.1007/BF01710710 - DOI
Publication types
MeSH terms
Substances
Grants and funding
LinkOut - more resources
Full Text Sources
Medical