Localized and Systemic Immune Responses against SARS-CoV-2 Following Mucosal Immunization - PubMed
- ️Fri Jan 01 2021
Localized and Systemic Immune Responses against SARS-CoV-2 Following Mucosal Immunization
Shaswath S Chandrasekar et al. Vaccines (Basel). 2021.
Abstract
The rapid transmission of SARS-CoV-2 in the USA and worldwide necessitates the development of multiple vaccines to combat the COVID-19 global pandemic. Previously, we showed that a particulate adjuvant system, quil-A-loaded chitosan (QAC) nanoparticles, can elicit robust immunity combined with plasmid vaccines when used against avian coronavirus. Here, we report on the immune responses elicited by mucosal homologous plasmid and a heterologous immunization strategy using a plasmid vaccine and a Modified Vaccinia Ankara (MVA) expressing SARS-CoV-2 spike (S) and nucleocapsid (N) antigens. Only the heterologous intranasal immunization strategy elicited neutralizing antibodies against SARS-CoV-2 in serum and bronchoalveolar lavage of mice, suggesting a protective vaccine. The same prime/boost strategy led to the induction of type 1 and type 17 T-cell responses and polyfunctional T-cells expressing multiple type 1 cytokines (e.g., IFN-γ, TNFα, IL-2) in the lungs and spleens of vaccinated mice. In contrast, the plasmid homologous vaccine strategy led to the induction of local mono and polyfunctional T-cells secreting IFN-γ. Outcomes of this study support the potential of QAC-nano vaccines to elicit significant mucosal immune responses against respiratory coronaviruses.
Keywords: COVID-19; SARS-CoV-2; heterologous vaccine; intranasal vaccine; nanovaccine.
Conflict of interest statement
A.M.T. and Y.P. have financial interests in Pan Genome Systems, Inc., a startup company developing animal and human vaccines.
Figures
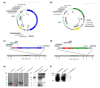
Design of SARS-CoV-2 vector vaccine constructs expressing S and N proteins. (a) Gene map of pCMV plasmid expressing Truncated S (TrS) protein. (b) Gene map of pCMV plasmid backbone expressing N protein. (c) MVA vaccine construct expressing TrS protein with the addition of C-terminal 6X His tag. (d) The MVA construct expressing N protein. All gene maps were generated using Snapgene software. (e) Western blot analysis with anti 6xHis-HRP antibody (left) and polyclonal mouse anti-SARS-CoV-2 spike sera (right) confirming expression of S protein from vaccine constructs. Lanes are as follows: Supernatant (lanes 2) HEK 293T cells transfected with control plasmid, supernatant (lanes 1 and 7) HEK 293T cells transfected with pCMV-TrS plasmid. Supernatant (lanes 3 and 6) from chicken embryonic fibroblast (CEF) cells infected with MVA-TrS and control non-infected supernatant (lane 4). Purified recombinant SARS-CoV-2 S Glycoprotein (BEI resources-NR-52396). (f) Western blot analysis with anti 6xHis-HRP antibody N from vaccine constructs. Lanes are as follows: Cell pellet (lane 3) HEK 293T cells transfected with control plasmid, cell pellet (lane 1) HEK 293T cells transfected with pCMV-N plasmid. Cell pellet (lane 2) from CEF cells infected with MVA-N.
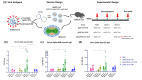
Generation of humoral immune responses in C57BL/6 mice following immunization with different vaccine constructs. (a) Outline for vaccine construct and immunization protocol using groups of C57BL/6 mice vaccinated with 3 doses of pQAC-CoV (I.N) or pQAC-CoV (I.M) with 3-week interval. Another group of C57BL/5 mice were vaccinated with pQAC-CoV (I.N) at week-0 followed by boost with MVA-CoV (I.N) at week-6. (b) ELISA titers of SARS-CoV-2 S-specific IgG in mice sera, (c) ELISA titers of SARS-CoV-2 spike receptor-binding domain (RBD)-specific IgG in mice serum and (d) ELISA titers of SARS-CoV-2 S-specific IgA in bronchoalveolar lavage (BAL), significance (*, p < 0.05, ****, p < 0.0001) was determined by two-way ANOVA. Data show mean ± SEM.
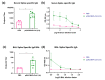
Heterologous vaccine strategy elicits spike-binding antibody responses. Groups of C57BL/5 mice were either unvaccinated (PBS) or immunized with pQAC/MVA-CoV (I.N). Serum and BAL samples were collected 3 weeks post boost. (a) SARS-CoV-2 spike-specific serum IgG binding endpoint titers measured by ELISA. (b) Serial serum dilutions of IgG binding to SARS-CoV-2 spike protein. (c) SARS-CoV-2 spike-specific BAL IgA binding endpoint titers measured by ELISA. (d) Serial BAL dilutions of IgA binding to SARS-CoV-2 spike protein. Significance (*, p < 0.05) was determined by one-way ANOVA. Data show mean ± SEM.
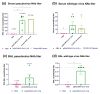
Heterologous vaccine strategy elicits SARS-CoV-2 neutralizing antibody responses. Groups of C57BL/5 mice were either unvaccinated (PBS) or immunized with pQAC/MVA-CoV (I.N). Serum and BAL samples were collected 3 weeks post boost. (a) 50% serum neutralization titer of pseudo-virus expressing SARS-CoV-2 spike, (b) serum neutralization titer of wild-type SARS-CoV-2, isolate USA-WA1/2020, (c) 50% BAL neutralization titer of pseudo-virus expressing SARS-CoV-2 spike, and (d) BAL neutralization titer of wild-type SARS-CoV-2, isolate USA-WA1/2020. Significance (*, p < 0.05; **, p < 0.01) was determined by one-way ANOVA. Data show mean ± SEM. The dotted line indicates the lower limit of detection.
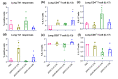
SARS-CoV-2 spike specific T cell responses in lungs of vaccinated C57BL/6 mice. Intracellular cytokine staining was performed on lungs harvested 3 weeks after final boost to assess T-cell responses. (a) Type 1 helper (Th1) responses (IFN-γ or TNFα or IL-2+), (b) type 2 helper (Th2) responses (IL-13+), (c) type 17 helper (Th17) responses (IL-17+), (d) type 1 cytotoxic (Tc1) responses (IFN-γ or TNFα or IL-2+), (e) type 2 cytotoxic (Tc2) responses (IL-13+), (f) type 17 cytotoxic (Tc17) responses (IL-17+) intracellular cytokine staining assays for lung T-cells in response to recombinant SARS-CoV-2 spike stimulation. Samples with lower live cells (<10,000) were excluded from analysis. Significance (**, p < 0.01) was determined by ANOVA compared to PBS controls. Data show mean ± SEM.
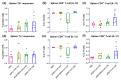
SARS-CoV-2 spike specific T cell responses in spleen of vaccinated C57BL/6 mice. Intracellular cytokine staining was performed on spleens harvested 3 weeks after final boost to assess T-cell responses. (a) Type 1 helper (Th1) responses (IFN-γ or TNFα or IL-2+), (b) type 2 helper (Th2) responses (IL-13+), (c) type 17 helper (Th17) responses (IL-17+), (d) type 1 cytotoxic (Tc1) responses (IFN-γ or TNFα or IL-2+), (e) type 2 cytotoxic (Tc2) responses (IL-13+), (f) type 17 cytotoxic (Tc17) responses (IL-17+) intracellular cytokine staining assays for spleen T-cells in response to recombinant SARS-CoV-2 spike stimulation. Significance (*, p < 0.05; **, p < 0.01) was determined by ANOVA compared to PBS controls. Data show mean ± SEM.
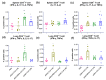
Immunization with SARS-CoV-2 vaccine constructs induces polyfunctional T cells. (a) CD4+ IFN-γ, TNFα, IL-2, and IL-17, (b) CD 4+ IL-2 and IL-17 (c) CD8+ IFN-γ, TNFα, IL-2, and IL-17 polyfunctional T-cells in the spleen of vaccinated mice. (d) CD4+ IFN-γ, TNFα, IL-2, and IL-17, (e) CD8+ IFN-γ and TNFα, (f) CD8+ IFN-γ, TNFα, and IL-17 polyfunctional T-cells in the lungs of vaccinated mice following intracellular cytokine staining assays for after recombinant SARS-CoV-2 spike stimulation. Significance (*, p < 0.05; **, p < 0.01) was determined by ANOVA compared to PBS controls. Data show mean ± SEM.
Similar articles
-
Pérez P, Astorgano D, Albericio G, Flores S, Sánchez-Cordón PJ, Luczkowiak J, Delgado R, Casasnovas JM, Esteban M, García-Arriaza J. Pérez P, et al. Front Immunol. 2022 Sep 12;13:995235. doi: 10.3389/fimmu.2022.995235. eCollection 2022. Front Immunol. 2022. PMID: 36172368 Free PMC article.
-
García-Arriaza J, Garaigorta U, Pérez P, Lázaro-Frías A, Zamora C, Gastaminza P, Del Fresno C, Casasnovas JM, Sorzano CÓS, Sancho D, Esteban M. García-Arriaza J, et al. J Virol. 2021 Mar 10;95(7):e02260-20. doi: 10.1128/JVI.02260-20. Epub 2021 Jan 7. J Virol. 2021. PMID: 33414159 Free PMC article.
-
Chandrasekar SS, Kingstad-Bakke BA, Wu CW, Phanse Y, Osorio JE, Talaat AM. Chandrasekar SS, et al. Vaccines (Basel). 2023 Jan 30;11(2):302. doi: 10.3390/vaccines11020302. Vaccines (Basel). 2023. PMID: 36851180 Free PMC article.
-
Chandrasekar SS, Phanse Y, Riel M, Hildebrand RE, Hanafy M, Osorio JE, Abdelgayed SS, Talaat AM. Chandrasekar SS, et al. Viruses. 2022 Jun 10;14(6):1262. doi: 10.3390/v14061262. Viruses. 2022. PMID: 35746733 Free PMC article.
-
Cavanagh D. Cavanagh D. Avian Pathol. 2003 Dec;32(6):567-82. doi: 10.1080/03079450310001621198. Avian Pathol. 2003. PMID: 14676007 Free PMC article. Review.
Cited by
-
Sun S, Li E, Zhao G, Tang J, Zuo Q, Cai L, Xu C, Sui C, Ou Y, Liu C, Li H, Ding Y, Li C, Lu D, Zhang W, Luo P, Cheng P, Gao Y, Tu C, Pitard B, Rosenecker J, Wang B, Liu Y, Zou Q, Guan S. Sun S, et al. Biomaterials. 2023 Jan;292:121907. doi: 10.1016/j.biomaterials.2022.121907. Epub 2022 Nov 18. Biomaterials. 2023. PMID: 36436305 Free PMC article.
-
Boulton S, Poutou J, Martin NT, Azad T, Singaravelu R, Crupi MJF, Jamieson T, He X, Marius R, Petryk J, Tanese de Souza C, Austin B, Taha Z, Whelan J, Khan ST, Pelin A, Rezaei R, Surendran A, Tucker S, Fekete EEF, Dave J, Diallo JS, Auer R, Angel JB, Cameron DW, Cailhier JF, Lapointe R, Potts K, Mahoney DJ, Bell JC, Ilkow CS. Boulton S, et al. Mol Ther. 2022 May 4;30(5):1885-1896. doi: 10.1016/j.ymthe.2021.10.008. Epub 2021 Oct 20. Mol Ther. 2022. PMID: 34687845 Free PMC article.
-
Hildebrand RE, Chandrasekar SS, Riel M, Touray BJB, Aschenbroich SA, Talaat AM. Hildebrand RE, et al. Microbiol Spectr. 2022 Oct 26;10(5):e0307522. doi: 10.1128/spectrum.03075-22. Epub 2022 Oct 6. Microbiol Spectr. 2022. PMID: 36200898 Free PMC article.
-
Optimized vaccine candidate MVA-S(3P) fully protects against SARS-CoV-2 infection in hamsters.
Abdelnabi R, Pérez P, Astorgano D, Albericio G, Kerstens W, Thibaut HJ, Coelmont L, Weynand B, Labiod N, Delgado R, Montenegro D, Puentes E, Rodríguez E, Neyts J, Dallmeier K, Esteban M, García-Arriaza J. Abdelnabi R, et al. Front Immunol. 2023 Oct 18;14:1163159. doi: 10.3389/fimmu.2023.1163159. eCollection 2023. Front Immunol. 2023. PMID: 37920464 Free PMC article.
-
Applications of Chitosan in Prevention and Treatment Strategies of Infectious Diseases.
Sinani G, Sessevmez M, Şenel S. Sinani G, et al. Pharmaceutics. 2024 Sep 13;16(9):1201. doi: 10.3390/pharmaceutics16091201. Pharmaceutics. 2024. PMID: 39339237 Free PMC article. Review.
References
-
- Zhou F., Yu T., Du R., Fan G., Liu Y., Liu Z., Xiang J., Wang Y., Song B., Gu X., et al. Clinical course and risk factors for mortality of adult inpatients with COVID-19 in Wuhan, China: A retrospective cohort study. Lancet. 2020;395:1054–1062. doi: 10.1016/S0140-6736(20)30566-3. - DOI - PMC - PubMed
Grants and funding
LinkOut - more resources
Full Text Sources
Other Literature Sources
Molecular Biology Databases
Miscellaneous