UV radiation affects antipredatory defense traits in Daphnia pulex - PubMed
- ️Wed Jan 01 2020
. 2020 Nov 21;10(24):14082-14097.
doi: 10.1002/ece3.6999. eCollection 2020 Dec.
Affiliations
- PMID: 33732430
- PMCID: PMC7771149
- DOI: 10.1002/ece3.6999
UV radiation affects antipredatory defense traits in Daphnia pulex
Franceen Eshun-Wilson et al. Ecol Evol. 2020.
Abstract
In aquatic environments, prey perceive predator threats by chemical cues called kairomones, which can induce changes in their morphology, life histories, and behavior. Predator-induced defenses have allowed for prey, such as Daphnia pulex, to avert capture by common invertebrate predators, such as Chaoborus sp. larvae. However, the influence of additional stressors, such as ultraviolet radiation (UVR), on the Daphnia-Chaoborus interaction is not settled as UVR may for instance deactivate the kairomone. In laboratory experiments, we investigated the combined effect of kairomones and UVR at ecologically relevant levels on induced morphological defenses of two D. pulex clones. We found that kairomones were not deactivated by UVR exposure. Instead, UVR exposure suppressed induced morphological defense traits of D. pulex juveniles under predation threat by generally decreasing the number of neckteeth and especially by decreasing the size of the pedestal beneath the neckteeth. UVR exposure also decreased the body length, body width, and tail spine length of juveniles, likely additionally increasing the vulnerability to Chaoborus predation. Our results suggest potential detrimental effects on fitness and survival of D. pulex subject to UVR stress, with consequences on community composition and food web structure in clear and shallow water bodies.
Keywords: Bayesian statistics; Chaoborus; Kairomone; MCMC chains; UV‐A; aquatic ecosystem; phenotypic plasticity; predator–prey interaction; zooplankton.
© 2020 The Authors. Ecology and Evolution published by John Wiley & Sons Ltd.
Conflict of interest statement
None declared.
Figures
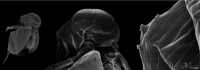
Scanning electron microscopy (SEM) images showing a 2nd instar juvenile male of the Daphnia pulex clone P5 that has been exposed to kairomones of Chaoborus flavicans larvae. Image includes full body, headshot, and close‐up of three induced neckteeth with pedestal of score type B. Photo‐credit: Jannicke Wiik‐Nielsen, Norwegian Veterinary Institute
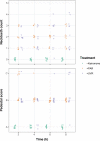
Number of neckteeth and pedestal score in 2nd instar offspring of Daphnia pulex mothers of clone UNI. In the UVR and PAR treatment, media‐filled jars with added kairomone extract have been exposed to UVR and PAR, respectively, for 2, 4, 6, and 8 hr before placing D. pulex mothers in the jars. In the control treatment “–Kairomone”, D. pulex mothers were kept in jars in the dark without addition of kairomone extract. Neckteeth count and pedestal scores of individual offspring juveniles (n = 10 per treatment group and time interval) are shown. A: No pedestal, B: small pedestal, C: large pedestal
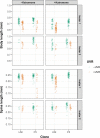
Body length and tail spine (spina) length of two Daphnia pulex clones (UNI, P5) in instar 1 and instar 2 juveniles with and without exposure to kairomone, and with exposure to UVR (+UVR) and with exposure to PAR (–UVR). There are no data available for clone P5 instar 1 juveniles in the +kairomone/+UVR treatment
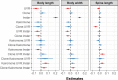
Summary of the predictor (“fixed”) effects for the final (reduced) three‐variate model of body length, body width, and spina length. All available data from both clones and instars were used. The thick and thin lines describe the 50% and 95% credible intervals (CI) of each parameter, respectively. Effects were considered strong (indicated in red) when their 95% CI did not include 0
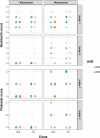
Number of neckteeth and pedestal score of two Daphnia pulex clones (UNI, P5) in instar 1 and instar 2 juveniles with and without exposure to kairomone, and with exposure to UVR (+UVR) and with exposure to PAR (–UVR). A: No pedestal, B: small pedestal, C: large pedestal
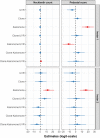
Summary of the predictor (“fixed”) effects for the final (reduced) bivariate model of neckteeth count and pedestal score for instar 1 and instar 2. All available data from both clones and instars were used; however, separate models were fit for each instar. The thick and thin lines describe the 50% and 95% credible intervals (CI) of each parameter, respectively. Effects were considered strong (indicated in red) when their 95% CI did not include 0
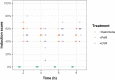
Neckteeth induction score (%) in 2nd instar offspring of Daphnia pulex mothers of clone UNI. In the UVR and PAR treatment, media‐filled jars with added kairomone extract have been exposed to UVR and PAR, respectively, for 2, 4, 6, and 8 hours before placing D. pulex mothers in the jars. In the control treatment ‘–Kairomone’, D. pulex mothers were kept in jars in the dark without addition of kairomone extract. Data of individual offspring juveniles (n = 10 per treatment group and time interval) are shown. A: no pedestal, B: small pedestal, C: large pedestal
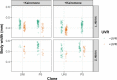
Body width of two Daphnia pulex clones (UNI, P5) in instar 1 and instar 2 juveniles with and without exposure to kairomone, and with exposure to UVR (+UVR) and with exposure to PAR (–UVR). There are no data available for clone P5 instar 1 juveniles in the +kairomone treatment
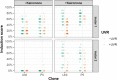
Neckteeth induction score of two Daphnia pulex clones (UNI, P5) in instar 1 and instar 2 juveniles with and without exposure to kairomone, and with exposure to UVR (+UVR) and with exposure to PAR (–UVR)
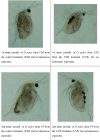
Comparison of ‘healthy’ animals that were exposed to PAR (i.e., no UVR) with animals from the UVR exposure treatment that showed signs of lipid store damage
Similar articles
-
Sperfeld E, Nilssen JP, Rinehart S, Schwenk K, Hessen DO. Sperfeld E, et al. Oecologia. 2020 Mar;192(3):687-698. doi: 10.1007/s00442-019-04588-6. Epub 2020 Jan 16. Oecologia. 2020. PMID: 31950263 Free PMC article.
-
Weiss LC, Kruppert S, Laforsch C, Tollrian R. Weiss LC, et al. PLoS One. 2012;7(5):e36879. doi: 10.1371/journal.pone.0036879. Epub 2012 May 9. PLoS One. 2012. PMID: 22590631 Free PMC article.
-
Influence of Cat Odor on Reproductive Behavior and Physiology in the House Mouse: (Mus Musculus).
Voznessenskaya VV. Voznessenskaya VV. In: Mucignat-Caretta C, editor. Neurobiology of Chemical Communication. Boca Raton (FL): CRC Press/Taylor & Francis; 2014. Chapter 14. In: Mucignat-Caretta C, editor. Neurobiology of Chemical Communication. Boca Raton (FL): CRC Press/Taylor & Francis; 2014. Chapter 14. PMID: 24830030 Free Books & Documents. Review.
-
Sensory Ecology of Predator-Induced Phenotypic Plasticity.
Weiss LC. Weiss LC. Front Behav Neurosci. 2019 Jan 18;12:330. doi: 10.3389/fnbeh.2018.00330. eCollection 2018. Front Behav Neurosci. 2019. PMID: 30713490 Free PMC article. Review.
Cited by
-
A predictive timeline of wildlife population collapse.
Cerini F, Childs DZ, Clements CF. Cerini F, et al. Nat Ecol Evol. 2023 Mar;7(3):320-331. doi: 10.1038/s41559-023-01985-2. Epub 2023 Jan 26. Nat Ecol Evol. 2023. PMID: 36702859 Review.
-
Neale PJ, Williamson CE, Banaszak AT, Häder DP, Hylander S, Ossola R, Rose KC, Wängberg SÅ, Zepp R. Neale PJ, et al. Photochem Photobiol Sci. 2023 May;22(5):1093-1127. doi: 10.1007/s43630-023-00370-z. Epub 2023 May 2. Photochem Photobiol Sci. 2023. PMID: 37129840 Free PMC article.
References
-
- Bais, A. F. , Lucas, R. M. , Bornman, J. F. , Williamson, C. E. , Sulzberger, B. , Austin, A. T. , Wilson, S. R. , Andrady, A. L. , Bernhard, G. , McKenzie, R. L. , Aucamp, P. J. , Madronich, S. , Neale, R. E. , Yazar, S. , Young, A. R. , de Gruijl, F. R. , Norval, M. , Takizawa, Y. , Barnes, P. W. , … Heikkilä, A. M. (2018). Environmental effects of ozone depletion, UV radiation and interactions with climate change: UNEP Environmental Effects Assessment Panel, update 2017. Photochemical & Photobiological Sciences, 17(2), 127–179. 10.1039/c7pp90043k - DOI - PMC - PubMed
-
- Bashevkin, S. M. , Christy, J. H. , & Morgan, S. G. (2020). Adaptive specialization and constraint in morphological defences of planktonic larvae. Functional Ecology, 34, 217–228. 10.1111/1365-2435.13464 - DOI
-
- Beklioglu, M. , Cetin, A. G. , Zorlu, P. , & Ay‐Zog, D. (2006). Role of planktonic bacteria in biodegradation of fish‐exuded kairomone in laboratory bioassays of diel vertical migration. Archiv Für Hydrobiologie, 165, 89–104. 10.1127/0003-9136/2006/0165-0089 - DOI
LinkOut - more resources
Full Text Sources