Odontoblast TRPC5 channels signal cold pain in teeth - PubMed
- ️Fri Jan 01 2021
. 2021 Mar 26;7(13):eabf5567.
doi: 10.1126/sciadv.abf5567. Print 2021 Mar.
Pamela Sotelo-Hitschfeld 1 3 , Christine König 1 , Viktor Sinica 1 4 , Amanda Wyatt 5 , Zoltan Winter 1 , Alexander Hein 6 , Filip Touska 1 4 , Susanne Reinhardt 7 , Aaron Tragl 1 , Ricardo Kusuda 1 , Philipp Wartenberg 5 , Allen Sclaroff 8 , John D Pfeifer 9 , Fabien Ectors 10 , Andreas Dahl 7 , Marc Freichel 11 , Viktorie Vlachova 4 , Sebastian Brauchi 3 12 , Carolina Roza 2 , Ulrich Boehm 5 , David E Clapham 13 , Jochen K Lennerz 14 , Katharina Zimmermann 15
Affiliations
- PMID: 33771873
- PMCID: PMC7997515
- DOI: 10.1126/sciadv.abf5567
Odontoblast TRPC5 channels signal cold pain in teeth
Laura Bernal et al. Sci Adv. 2021.
Abstract
Teeth are composed of many tissues, covered by an inflexible and obdurate enamel. Unlike most other tissues, teeth become extremely cold sensitive when inflamed. The mechanisms of this cold sensation are not understood. Here, we clarify the molecular and cellular components of the dental cold sensing system and show that sensory transduction of cold stimuli in teeth requires odontoblasts. TRPC5 is a cold sensor in healthy teeth and, with TRPA1, is sufficient for cold sensing. The odontoblast appears as the direct site of TRPC5 cold transduction and provides a mechanism for prolonged cold sensing via TRPC5's relative sensitivity to intracellular calcium and lack of desensitization. Our data provide concrete functional evidence that equipping odontoblasts with the cold-sensor TRPC5 expands traditional odontoblast functions and renders it a previously unknown integral cellular component of the dental cold sensing system.
Copyright © 2021 The Authors, some rights reserved; exclusive licensee American Association for the Advancement of Science. No claim to original U.S. Government Works. Distributed under a Creative Commons Attribution NonCommercial License 4.0 (CC BY-NC).
Figures
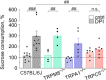
Percent change in sucrose consumption relative to baseline (dashed line) after DPI. DPI enhanced the consumption of 5% room temperature sucrose water to 293 ± 46% above baseline (###P = 0.00001). Lack of TRPC5 (##P = 0.005; 171 ± 21%), but not TRPM8 (P = 0.9; 299 ± 34%) or TRPA1 (P = 0.1; 225 ± 43%), reverts glucose consumption after DPI to baseline. The reduction in sucrose consumption in TRPC5−/− (n.s. P = 0.5), but not TRPA1−/− (##P = 0.006) or TRPM8−/− (##P = 0.004), was not different from the respective controls without DPI. n.s., not significant.
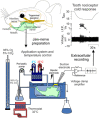
(Top) Illustration of mouse head with jaws and their innervation. The mandible-inferior alveolar nerve preparation is derived from the lower jaw and transferred to an organ bath consisting of an external solution and a mini-tube that is connected to a temperature-controlled application system. Connection of the application system’s heating coil to a heating/cooling thermostat board permits rapid exchange of solution temperature in the mini-tube, where the preparation is exposed to chemical compounds and cold temperatures. Both the external and internal bath are supplied with oxygenized extracellular solution. Because of the short length of the inferior alveolar nerve, suction electrodes from glass capillaries are applied and the amplifier is used in a single-ended configuration. The external bath is required to prevent cold block in the nerve when the teeth in the mini-tube are exposed to cold. Action potentials from the inferior alveolar nerve are recorded in gap-free mode with Spike 2 (Materials and Methods).
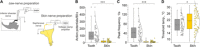
(A) Schematic illustration of extracellular recordings from jaw-nerve as compared to skin-nerve preparations.(B to D) Comparison of the (B) cold response magnitude, (C) peak frequency, and (D) threshold temperature of C57BL/6J teeth (n = 45) and skin (n = 59) nociceptors. Statistical significance was identified by a two-sided Student’s t test: ***P = 2 × 10−8, ***P = 3 × 10−11, and *P = 0.05. Skin nociceptor cold responses are from (6, 50) and refer to the same background strain at equivalent stimulus conditions.
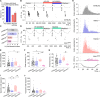
(A) Percent cold-sensitive tooth nociceptors blocked by HC-030031 (n = 9 of 9 fibers) and ML204/HC-070 (n = 6 of 13 fibers) and respective fraction of block (means ± SEM). (B) C57BL/6J wt tooth nociceptor recording with temperature (top) and instantaneous frequency pattern (I.F.P.; bottom) blocked by HC-070 and HC-030031. Circles represent action potentials. (C) Tooth cold responses in TRPA1−/− (n = 10 of 138, P = 1.0), TRPC5−/− (n = 8 of 217, ‡P = 0.04), and TRPC5/A1-DKO (n = 5 of 177, ‡P = 0.02), chi-square tests versus C57BL/6J (n = 45 of 570). (D) Typical cold response of a TRPC5/A1-DKO tooth nociceptor with temperature (top) and instantaneous frequency pattern (I.F.P.; bottom). As in (B), circles represent action potentials, and horizontal bars and arrows indicate applications and respective time intervals. (E to G) Teeth nociceptor cold response characteristics according to genotype, (E) cold response magnitude [P = 0.2 between groups by one-way analysis of variance (ANOVA)], (F) peak firing frequency (P = 0.01), and (G) temperature threshold (P = 0.06). TRPC5/A1-DKO were statistically different from C57BL/6J wt (#P = 0.04 and #P = 0.02) and TRPC5−/− (#P = 0.05, ##P = 0.001, and ##P = 0.007). TRPC5−/− were statistically different from TRPA1−/− (#P = 0.03) and C57BL/6J (#P = 0.05) in least significant difference post hoc tests. (H to J) Histograms in bins of 2 s (H) and respective box plots of (I) dynamic and (J) constant cold responses. Significant differences between groups by one-way ANOVA existed for dynamic (10 to 42 s of the histogram; P = 0.01) but not constant cold (44 to 80 s of the histogram; P = 0.6). TRPC5/A1-DKO (n = 5) and TRPA1−/− (n = 10) were different from C57BL/6J (#P = 0.02 and #P = 0.03, n = 45) and TRPC5−/− (#P = 0.01 and ##P = 0.02, n = 8). Histograms present data as means ± SEM, and asterisks refer to two-sided Student’s t test comparison of respective knockouts with the C57BL/6J background strain (3 × 10−6 < *P < 0.002). Lines represent medians, squares represent the mean, boxes represent the interquartile range (IQR), and whiskers represent 2.2-fold of IQR after exclusion of the >2.2 IQR outliers identified by crosses.
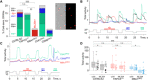
(A) Characterization and quantification of cold-sensitive cultured DPANs (C57BL/6J: n = 23 of 136) based on Ca2+ transients measured with Fura-2 AM and sensitivity to TRP channel modulators menthol (me, n = 7), carvacrol (crv, n = 3), both (n = 10, descending diagonal stripes), neither (n = 4, ascending diagonal stripes), and riluzole (rlz, n = 1). Cold-sensitive DPANs insensitive to menthol and carvacrol (ascending diagonal stripes) are unchanged in TRPC5−/− (n = 1 of 14 in 93) versus C57BL/6J (3 of 23 in 136; n.s. P = 0.86), but increased in TRPM8A1-DKO (n = 23 in 457; ‡‡P = 0.002 and ‡‡‡P = 0.00003). In TRPC5A1-DKO, cold-sensitive cells (n = 20 of 100) were mostly menthol sensitive (n = 15; crv+ n = 10; both, n = 7 and none, n = 2). TRPC5M8-DKO cold-sensitive cells (n = 5 of 103) were mostly sensitive to carvacrol (n = 3; me+ n = 2; both, n = 1 and none, n = 1). Inset: Photomicrograph of cultured mouse TG neurons with red DiI retro-labeled DPANs. (B and C) Ca2+ transient traces from (B) C57BL/6J representative of four types of cold-sensitive neurons and (C) TRPM8A1-DKO (red), TRPC5A1-DKO (green), and TRPC5M8-DKO (blue). Bottom: Temperature stimulator command. (D) TRPM8/A1-DKO control (cntrl) cold responses (n = 23) were smaller than in C57BL/6J (n = 23; ###P = 0.0007) but not in TRPC5−/− neurons (n = 13; P = 0.2). ML204 (circles = treated neurons) blocked most cold responses in TRPM8/A1-DKO (≥50% block = filled circles, n = 12; *P = 0.01) and some in C57BL/6J neurons (n = 15; *P = 0.02), but not in TRPC5−/− neurons (n = 13; n.s. P = 0.2). n.s., not significant. Lines are medians, squares mean, boxes IQR, whiskers 2.2-fold IQR after exclusion of >2.2 IQR outliers identified by crosses.
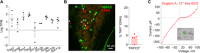
(A) Relative expression of TRP channel genes and nociceptor-specific subtypes of voltage-gated sodium channel genes in mouse DPANs given as transcripts per million (TPM). Data are presented as means ± SEM. Dots represent each replicate. Replicates with value 0 are not represented in the logarithmic scale. TRPC5 was not among the transcripts (see table S1). (B) Red DPANs (arrowheads) in TRPC5 reporter mouse ganglion (TG) multiphoton stacks of maxillo-mandibular regions. A total of 176 DPANs had 11 TRPC5+ neurons (seven TGs, five mice). (C) Typical doubly rectifying current-voltage relation observed in a cultured TRPC5+ neuron of a reporter mouse (n = 13). TRPC5 current is small and is only identified as Englerin-sensitized current subtracted from the baseline current in the absence of chloride in the solution.
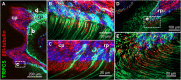
(A) TRPC5 reporter mouse molar tooth whole mount with densely packed TRPC5+ odontoblasts at the pulp-dentin boundary. (B and C) In tight association with sensory nerves. Green, TRPC5; red, βIII-tubulin; circle indicates area shown in (D) and (E) from a subsequent section. cp, coronal pulp; rp, radicular pulp. (E) Oblique section through the predentinal radicular pulp to visualize the tight association of TRPC5+ odontoblast processes with their sensory nerves.
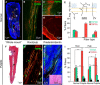
(A) Whole-mount panography of a human tooth; regions evaluated for TRPC5 expression are indicated; dotted line, level used in root measurement. (B) TRPC5+ nerve fibers in the radicular pulp double labeled with TRPM8. (C) TRPC5+ nerve fibers double labeled with PGP9.5 within the inner third of the dentinal tubules (type IV) ~40 μm above the odontoblast-predentinal border. (D) TRPC5+ branched fibers (type II/III) in the predentin/dentin layer. (E) Quantification of TRPC5+ and TRPM8+ fiber types (four teeth). TRPC5/PGP9.5 and TRPM8/PGP9.5 for type I: 13/51 and 7/22; type II/III: 41/70 and 0/35; type IV: 26/105 and 6/49. (F) Hematoxylin and eosin (H&E)–stained human tooth whole mount with degenerated dentin (caries) and pulpitis. (G) Abundant TRPC5+ and decreased TRPM8+ (arrows) nerve fibers in the pulpitic tooth root. (H) TRPC5+ nerve fibers (type IV) in degenerating dentin (inset, H&E). (I) Increased TRPC5+ (190 of 660 versus 394 of 607 fibers; **P = 0.002), decreased TRPM8+ (197 of 660 versus 67 of 607; **P = 0.005), and similar proportions of colabeled fibers (black) in normal versus pulpitic tooth roots [132 ± 78 (n = 4) versus 86 ± 21 (n = 7; P = 0.2)] and in the tooth pulp [TRPC5+: 15 of 51 versus 81 of 161; *P = 0.01; TRPM8+: 622 of 161 versus 9 of 22; **P = 0.008; colabeled fibers (black): 12 ± 3 (n = 4) versus 18 ± 8 (n = 9); P = 0.3]. Error bars, SEM.
Similar articles
-
El Karim IA, Linden GJ, Curtis TM, About I, McGahon MK, Irwin CR, Lundy FT. El Karim IA, et al. Pain. 2011 Oct;152(10):2211-2223. doi: 10.1016/j.pain.2010.10.016. Epub 2010 Dec 17. Pain. 2011. PMID: 21168271
-
Intercellular signal communication among odontoblasts and trigeminal ganglion neurons via glutamate.
Nishiyama A, Sato M, Kimura M, Katakura A, Tazaki M, Shibukawa Y. Nishiyama A, et al. Cell Calcium. 2016 Nov;60(5):341-355. doi: 10.1016/j.ceca.2016.07.003. Epub 2016 Jul 15. Cell Calcium. 2016. PMID: 27452727
-
TRPA1 and TRPV4 activation in human odontoblasts stimulates ATP release.
Egbuniwe O, Grover S, Duggal AK, Mavroudis A, Yazdi M, Renton T, Di Silvio L, Grant AD. Egbuniwe O, et al. J Dent Res. 2014 Sep;93(9):911-7. doi: 10.1177/0022034514544507. Epub 2014 Jul 25. J Dent Res. 2014. PMID: 25062738 Free PMC article.
-
Zholos AV. Zholos AV. Handb Exp Pharmacol. 2014;222:129-56. doi: 10.1007/978-3-642-54215-2_6. Handb Exp Pharmacol. 2014. PMID: 24756705 Review.
-
Odontoblast: a mechano-sensory cell.
Magloire H, Couble ML, Thivichon-Prince B, Maurin JC, Bleicher F. Magloire H, et al. J Exp Zool B Mol Dev Evol. 2009 Jul 15;312B(5):416-24. doi: 10.1002/jez.b.21264. J Exp Zool B Mol Dev Evol. 2009. PMID: 19097166 Review.
Cited by
-
Thermosensing ability of TRPC5: current knowledge and unsettled questions.
Ptakova A, Vlachova V. Ptakova A, et al. J Physiol Sci. 2024 Oct 3;74(1):50. doi: 10.1186/s12576-024-00942-3. J Physiol Sci. 2024. PMID: 39363236 Free PMC article. Review.
-
Li YY, Lv QY, Zheng GT, Liu D, Ma J, He GM, Zhang LB, Zheng S, Li HP, Pan YH. Li YY, et al. Zool Res. 2022 Jan 18;43(1):52-63. doi: 10.24272/j.issn.2095-8137.2021.209. Zool Res. 2022. PMID: 34821086 Free PMC article.
-
Ion channels of cold transduction and transmission.
Lewis CM, Griffith TN. Lewis CM, et al. J Gen Physiol. 2024 Oct 7;156(10):e202313529. doi: 10.1085/jgp.202313529. Epub 2024 Jul 25. J Gen Physiol. 2024. PMID: 39051992 Free PMC article. Review.
-
Ohyama S, Ouchi T, Kimura M, Kurashima R, Yasumatsu K, Nishida D, Hitomi S, Ubaidus S, Kuroda H, Ito S, Takano M, Ono K, Mizoguchi T, Katakura A, Shibukawa Y. Ohyama S, et al. Front Physiol. 2022 Dec 14;13:891759. doi: 10.3389/fphys.2022.891759. eCollection 2022. Front Physiol. 2022. PMID: 36589456 Free PMC article.
-
Dentin Mechanobiology: Bridging the Gap between Architecture and Function.
Fu X, Kim HS. Fu X, et al. Int J Mol Sci. 2024 May 22;25(11):5642. doi: 10.3390/ijms25115642. Int J Mol Sci. 2024. PMID: 38891829 Free PMC article. Review.
References
-
- Kassebaum N. J., Bernabé E., Dahiya M., Bhandari B., Murray C. J. L., Marcenes W., Global burden of untreated caries: A systematic review and metaregression. J. Dent. Res. 94, 650–658 (2015). - PubMed
-
- Alghaithy R. A., Qualtrough A. J. E., Pulp sensibility and vitality tests for diagnosing pulpal health in permanent teeth: A critical review. Int. Endod. J. 50, 135–142 (2017). - PubMed
-
- Brännström M., Johnson G., Movements of the dentine and pulp liquids on application of thermal stimuli. An in vitro study. Acta Odontol. Scand. 28, 59–70 (1970). - PubMed
-
- Vriens J., Nilius B., Voets T., Peripheral thermosensation in mammals. Nat. Rev. Neurosci. 15, 573–589 (2014). - PubMed
Publication types
LinkOut - more resources
Full Text Sources
Other Literature Sources
Molecular Biology Databases