Biosynthesis and synthetic biology of psychoactive natural products - PubMed
- ️Fri Jan 01 2021
Review
Biosynthesis and synthetic biology of psychoactive natural products
Cooper S Jamieson et al. Chem Soc Rev. 2021.
Abstract
Psychoactive natural products play an integral role in the modern world. The tremendous structural complexity displayed by such molecules confers diverse biological activities of significant medicinal value and sociocultural impact. Accordingly, in the last two centuries, immense effort has been devoted towards establishing how plants, animals, and fungi synthesize complex natural products from simple metabolic precursors. The recent explosion of genomics data and molecular biology tools has enabled the identification of genes encoding proteins that catalyze individual biosynthetic steps. Once fully elucidated, the "biosynthetic pathways" are often comparable to organic syntheses in elegance and yield. Additionally, the discovery of biosynthetic enzymes provides powerful catalysts which may be repurposed for synthetic biology applications, or implemented with chemoenzymatic synthetic approaches. In this review, we discuss the progress that has been made toward biosynthetic pathway elucidation amongst four classes of psychoactive natural products: hallucinogens, stimulants, cannabinoids, and opioids. Compounds of diverse biosynthetic origin - terpene, amino acid, polyketide - are identified, and notable mechanisms of key scaffold transforming steps are highlighted. We also provide a description of subsequent applications of the biosynthetic machinery, with an emphasis placed on the synthetic biology and metabolic engineering strategies enabling heterologous production.
Conflict of interest statement
Conflicts of interest
The authors declare the following competing financial interest(s):
John Billingsley is an employee of Invizyne, Technologies (Monrovia, CA, USA), a company seeking to commercialize synthetic biochemistry.
Figures
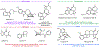
Four categories of psychoactive natural products or derivatives described in this review.
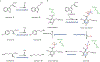
(A) three amino acids are decarboxylated to give primary amines that are building blocks for alkaloids; (B) mechanism of the PLP-dependent tryptophan decarboxylase
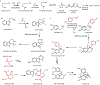
(A) formation of the pyrrolidine intermediate on pathway to tropane alkaloids; (B) the Pictet-Spengler reaction involving tryptamine to form tetrahydro-β-carboline intermediates; (C) the Pictet-Spengler reaction involving dopamine to form tetrahydroisoquinoline on pathway to morphine.
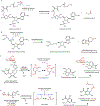
(A) acetyltransferase-catalyzed acetyltransfer; (B) methyltransferase-catalyzed methyl transfer; (C) glucosyltransferase-catalyzed glucosyl transfer.; and (D) prenyltransferase-catalyzed prenyl transfer.
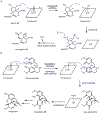
(A) secologanin synthase in biosynthesis of monoterpene indole alkaloids; (B) salutaridine synthase in biosynthesis of morphine family of opioids.
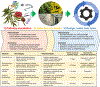
Strategies in synthetic biology.
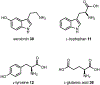
Amino acid building blocks for hallucinogens that target serotonin receptors.
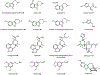
*Note that LSD 3 is a semisynthetic compound derived from lysergic acid (Section 2.5).
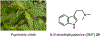
Image on the left courtesy of Paulo Pedro P. R. Costa via. CC-4.0.
https://upload.wikimedia.org/wikipedia/commons/0/01/PsychotriaviridisFrutoDSC75.jpg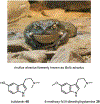
Image on top courtesy of Wildfeurer via. CC-3.0.
https://upload.wikimedia.org/wikipedia/commons/4/4f/2009-03-13Bufo_alvarius067.jpg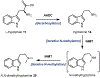
Biosynthesis of DMT.
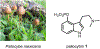
Image on left courtesy of Alan Rockefeller via CC-3.0.
https://upload.wikimedia.org/wikipedia/commons/4/46/Psilocybe_mexicana_53960.jpg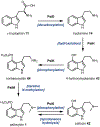
Biosynthetic pathway of psilocybin and psilocin from
l-tryptophan.
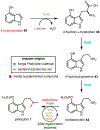
Engineered production of psilocybin in E. coli.
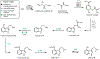
Engineered production of psilocybin and psilocin in yeast.
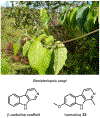
Image on left courtesy Forest and Kim Starr via CC-2.0.
https://upload.wikimedia.org/wikipedia/commons/1/17/Starr-140222-0335-Banisteriopsis_caapi-leaves-Haiku-Maui_%2825240510635%29.jpg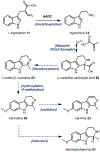
Proposed biosynthesis of harmala alkaloids.
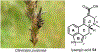
Image on the left courtesy of Bildoj via CC-3.0.
https://upload.wikimedia.org/wikipedia/commons/c/c4/Dactylis_026.JPG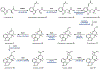
Biosynthesis of lysergic acid from
l-tryptophan.
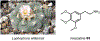
Image on the left courtesy of Peter A. Mansfeld via CC-3.0.
https://upload.wikimedia.org/wikipedia/commons/6/69/Lophophora_williamsii_pm.jpg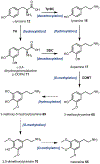
Proposed biosynthesis of mescaline.
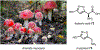
Amanita muscaria contains about ~100–1000 ppm of ibotenic acid and muscimol.
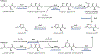
Biosynthesis of ibotenic acid and muscimol from
l-glutamic acid.
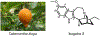
Image courtesy of Christian Kunath via CC-3.0.
https://twitter.com/sesamothamnus/status/1031998713760231424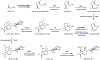
Biosynthesis of secologanin from geranyl pyrophosphate (GPP).
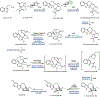
Biosynthesis of ibogaine from tryptamine and secologanin.
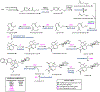
Heterologous production of strictosidine in S. cerevisiae.
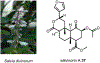
Salvia divinorum contains salvinorin A, a structurally unique terpene hallucinogen. Image on the left courtesy of Eric Hunt via CC-2.5.
https://upload.wikimedia.org/wikipedia/commons/3/35/Salvia_divinorum_-1.jpg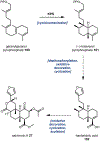
Proposed biosynthetic pathway for salvinorin A.
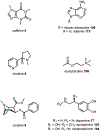
Alkaloidal stimulants as structural mimics of neurotransmitters.
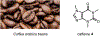
Coffea arabica (the dominant coffee cultivar) contains ~1.2 percent dry weight caffeine.
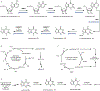
(A) Major caffeine biosynthetic route identified in Camellia sinensis and Coffea arabica. (B) SAH-derived adenosine may be funneled into purine metabolism in tea leaves following methyl transfer. (C) Xanthine recycle pathway utilized during heterologous production in yeast. (D) Novel xanthine-to-caffeine conversion pathway leveraged for caffeine production in E. coli.
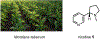
Nicotiana tabacum leaves contain 2 to 8 percent dry weight nicotine.
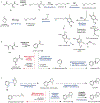
(A) N-methylpyrrolinium formation via the polyamine pathway. (B) Proposed reduction of nicotinic acid via A622. (C) Proposed oxidation of condensation products via BBL towards nicotine, nornicotine.
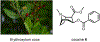
Image on left courtesy of Danna Lizeth Guevara Prieto via CC-4.0.
https://www.inaturalist.org/photos/22483426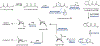
Formation of tropine, pseudotropine.
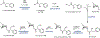
Scopolamine biosynthesis from phenylalanine and tropine.
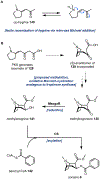
(A) Racemization of the cocaine pathway intermediate decarboxylation product hygrine. (B) Proposed biosynthesis of methylecognone and subsequent formation of cocaine.
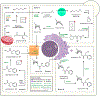
Production of tropane alkaloids in yeast.
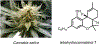
The Cannabis sativa plant typically contains 5–16% tetrahydrocannabinol (7).
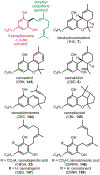
Structural motifs and examples of isolated natural products from the Cannabis plant.
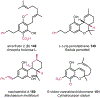
Structural deviations highlighted in red. Amorfrutin 2 (B) (148) is a 148 derivative, (–)-cis-perrottetinene (149) is a 7 derivative, machaeridiol (150) is a 8 derivative, and 6-chloro-cannabiorchichromene (151) is a 144 derivative.
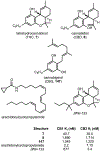
CB1 and CB2 activity for 7, 8, 147, the natural endocannabinoid arachidonylcyclopropylamide, and synthetic analogue JWH-133.
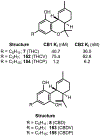
CB1 and CB2 activity of THC (7) with varying C3 alkyl chain lengths, propyl (varin, 152) and heptyl (phorol, 154). CBD alkyl chain length derivatives also shown for clarity.
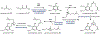
Biosynthesis of cannabigerol (147) and cannabinerol (159) from hexanoyl-CoA 156 and malonyl-CoA 127.
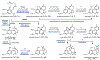
Biosynthesis of tetrahydrocannabinol (7), cannabidiol (8), cannabichromene (144), and further nonenzymatic derivatized products.
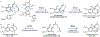
(A) Enzymatic dehydrogenation reaction leads to a reactive quinone methide intermediate 166 that can undergo various pericyclic reactions to yield all cannabis scaffolds. Flavin adenine dinucleotide (FAD), R = C16H26N5O13P2. (B) Related enzymatic transformations by CBCAS and CBDAS form CBCA (162) and CBDA (161)
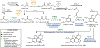
Heterologous production of tetrahydrocannabinolic acid (160) and cannabidiolic acid (161).
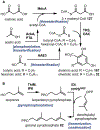
Cell-free system for improved olivetolic acid, divarinic acid, and geranyl pyrophosphate production.
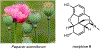
On average, poppy bulbs contain 16% by weight morphine 9.
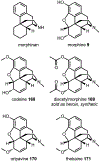
Structures of natural morphinan opioids and synthetic compound diacetylmorphine (heroin, 169).
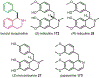
Structures of simple benzyl isoquinolines that play key roles in opioid biosynthesis (172, 28, 27) and as antispasmodic drugs (173).
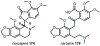
Phthalide isoquinoline opioid natural products, noscapine (174) and narceine (175).
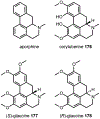
Aporphine opioids corytuberine (176), natural (S)-glaucine (177) and unnatural (R)-glaucine (178).
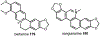
Examples of berberine opioids, berberine (179) and sanguinarine (180).
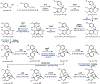
Biosynthesis of the morphine opioids from dopamine 17 and 4-hydroxyphenylacetaldehyde 26.
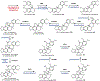
Noscapine biosynthesis from (S)-reticuline.
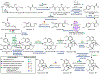
Heterologous production of thebaine and hydrocodone from sugar in yeast.
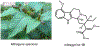
Image on left courtesy of Thor Porre via CC-3.0.
https://commons.wikimedia.org/wiki/File:Kratom_tree.jpg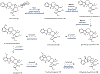
Proposed biosynthetic route to kratom alkaloids.
Similar articles
-
Toward the Heterologous Biosynthesis of Plant Natural Products: Gene Discovery and Characterization.
Wang H, Guo H, Wang N, Huo YX. Wang H, et al. ACS Synth Biol. 2021 Nov 19;10(11):2784-2795. doi: 10.1021/acssynbio.1c00315. Epub 2021 Nov 10. ACS Synth Biol. 2021. PMID: 34757715 Review.
-
Metabolic engineering for the production of natural products.
Pickens LB, Tang Y, Chooi YH. Pickens LB, et al. Annu Rev Chem Biomol Eng. 2011;2:211-36. doi: 10.1146/annurev-chembioeng-061010-114209. Annu Rev Chem Biomol Eng. 2011. PMID: 22432617 Free PMC article. Review.
-
A pharmaceutical model for the molecular evolution of microbial natural products.
Fewer DP, Metsä-Ketelä M. Fewer DP, et al. FEBS J. 2020 Apr;287(7):1429-1449. doi: 10.1111/febs.15129. Epub 2019 Nov 22. FEBS J. 2020. PMID: 31693795 Review.
-
Engineered Biosynthesis of Medicinally Important Plant Natural Products in Microorganisms.
Zhang S, Wang S, Zhan J. Zhang S, et al. Curr Top Med Chem. 2016;16(15):1740-54. doi: 10.2174/1568026616666151012112637. Curr Top Med Chem. 2016. PMID: 26456465 Review.
-
Recent advances in combinatorial biosynthesis for drug discovery.
Sun H, Liu Z, Zhao H, Ang EL. Sun H, et al. Drug Des Devel Ther. 2015 Feb 12;9:823-33. doi: 10.2147/DDDT.S63023. eCollection 2015. Drug Des Devel Ther. 2015. PMID: 25709407 Free PMC article. Review.
Cited by
-
Liu H, Jiang H, Liu X, Wang X. Liu H, et al. Chem Biomed Imaging. 2023 Mar 30;1(9):767-784. doi: 10.1021/cbmi.3c00021. eCollection 2023 Dec 25. Chem Biomed Imaging. 2023. PMID: 39473839 Free PMC article. Review.
-
Leveraging yeast to characterize plant biosynthetic gene clusters.
Wu Y, Gong FL, Li S. Wu Y, et al. Curr Opin Plant Biol. 2023 Feb;71:102314. doi: 10.1016/j.pbi.2022.102314. Epub 2022 Nov 30. Curr Opin Plant Biol. 2023. PMID: 36463029 Free PMC article. Review.
-
Ergotamine Stimulates Human 5-HT4-Serotonin Receptors and Human H2-Histamine Receptors in the Heart.
Jacob H, Braekow P, Schwarz R, Höhm C, Kirchhefer U, Hofmann B, Neumann J, Gergs U. Jacob H, et al. Int J Mol Sci. 2023 Mar 1;24(5):4749. doi: 10.3390/ijms24054749. Int J Mol Sci. 2023. PMID: 36902177 Free PMC article.
-
Engineered Production of Strictosidine and Analogues in Yeast.
Misa J, Billingsley JM, Niwa K, Yu RK, Tang Y. Misa J, et al. ACS Synth Biol. 2022 Apr 15;11(4):1639-1649. doi: 10.1021/acssynbio.2c00037. Epub 2022 Mar 16. ACS Synth Biol. 2022. PMID: 35294193 Free PMC article.
-
The protective effect of natural medicines in rheumatoid arthritis via inhibit angiogenesis.
Gao C, Song XD, Chen FH, Wei GL, Guo CY. Gao C, et al. Front Pharmacol. 2024 May 31;15:1380098. doi: 10.3389/fphar.2024.1380098. eCollection 2024. Front Pharmacol. 2024. PMID: 38881875 Free PMC article. Review.
References
-
- Merlin MD, Econ. Bot, 2003, 57, 295–323.
-
- Guerra-Doce E, Time Mind, 2015, 8, 91–112.
-
- Buenz EJ, Verpoorte R and Bauer BA, Annu. Rev. Pharmacol. Toxicol, 2018, 58, 509–530. - PubMed
-
- Efferth T, Banerjee M, Paul NW, Abdelfatah S, Arend J, Elhassan G, Hamdoun S, Hamm R, Hong C, Kadioglu O, Naß J, Ochwangi D, Ooko E, Ozenver N, Saeed MEM, Schneider M, Seo EJ, Wu CF, Yan G, Zeino M, Zhao Q, Abu-Darwish MS, Andersch K, Alexie G, Bessarab D, Bhakta-Guha D, Bolzani V, Dapat E, Donenko FV, Efferth M, Greten HJ, Gunatilaka L, Hussein AA, Karadeniz A, Khalid HE, Kuete V, Lee IS, Liu L, Midiwo J, Mora R, Nakagawa H, Ngassapa O, Noysang C, Omosa LK, Roland FH, Shahat AA, Saab A, Saeed EM, Shan L and Titinchi SJJ, Phytomedicine, 2016, 23, 166–173. - PubMed
-
- George JR, Michaels TI, Sevelius J and Williams MT, J. Psychedelic Stud, 2019, 4, 4–15.
Publication types
MeSH terms
Substances
LinkOut - more resources
Full Text Sources
Other Literature Sources