Beyond ribose and phosphate: Selected nucleic acid modifications for structure-function investigations and therapeutic applications - PubMed
- ️Fri Jan 01 2021
Review
. 2021 Apr 28:17:908-931.
doi: 10.3762/bjoc.17.76. eCollection 2021.
Affiliations
- PMID: 33981365
- PMCID: PMC8093555
- DOI: 10.3762/bjoc.17.76
Review
Beyond ribose and phosphate: Selected nucleic acid modifications for structure-function investigations and therapeutic applications
Christopher Liczner et al. Beilstein J Org Chem. 2021.
Abstract
Over the past 25 years, the acceleration of achievements in the development of oligonucleotide-based therapeutics has resulted in numerous new drugs making it to the market for the treatment of various diseases. Oligonucleotides with alterations to their scaffold, prepared with modified nucleosides and solid-phase synthesis, have yielded molecules with interesting biophysical properties that bind to their targets and are tolerated by the cellular machinery to elicit a therapeutic outcome. Structural techniques, such as crystallography, have provided insights to rationalize numerous properties including binding affinity, nuclease stability, and trends observed in the gene silencing. In this review, we discuss the chemistry, biophysical, and structural properties of a number of chemically modified oligonucleotides that have been explored for gene silencing.
Keywords: antisense; chemically modified oligonucleotides; crystallography; siRNA; structure.
Copyright © 2021, Liczner et al.
Figures
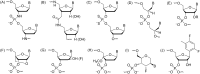
Structures of the chemically modified oligonucleotides (A) N3' → P5' phosphoramidate linkage, (B) amide (AM1) linkage, (C) phosphorodithioate (PS2), (D) glycol nucleic acid (R-isomer), (E) 2'-O-alkyl modifications (R = -CH3, -CH2CH2OCH3), (F) locked nucleic acids (LNA)/bridged nucleic acids (BNA), (G) arabinose (ANA) and arabinofluoro (FANA) nucleic acids, (H) C4'-modified nucleic acids, (I) 3'-fluorohexitol nucleic acid, (J) ribo-difluorotoluyl-modified nucleic acid.
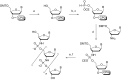
Synthesis of a N3' → P5' phosphoramidate linkage by solid-phase synthesis. (a) dichloroacetic acid; (b) ClP(NiPr2)(OCE); (c) tetrazole/water; (d) triethylamine/carbon tetrachloride; (e) repeat steps a–d; (f) detritylate then deprotect with NH3. DMT = dimethoxytrityl, CPG = succinyl-linked long chain alkylamine controlled pore glass solid support, CE = 2-cyanoethyl. Adapted from [64].
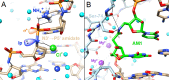
Crystal structures of (A) N3' → P5' phosphoramidate DNA (PDB ID 363D) [71] and (B) amide (AM1) RNA in complex with Bacillus halodurans RNase H (PDB ID 5VAJ) [73]. The relative orientation of the N3' n and P–O5' σ* orbitals in the backbone of 3'-NP DNA are consistent with an anomeric effect. The 3'-nitrogen is H-bonded to a chloride anion (green sphere) and the phosphate group forms a salt bridge to ammonium. Water molecules are cyan spheres and H-bonds are drawn with thin lines.
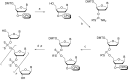
Synthesis of a phosphorodithioate linkage by solid-phase synthesis. (a) detritylation; (b) tetrazole; (c) sulfurization, capping, then washing; (d) repeat steps a–c; (e) detritylate then deprotect with NH4OH. R = pyrrolidino, R' = β-thiobenzoylethyl. Adapted from [85].
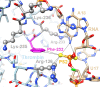
Close-up view of a key interaction between the PS2-modified antithrombin RNA aptamer and thrombin in the crystal structure of the complex (PDB ID 5DO4) [95]. An RNA-induced fit brings the PS2 moiety in close contact with the edge of Phe-232 (magenta carbon atoms) that forms a hydrophobic patch surrounded by four basic residues (side chains highlighted in ball-and-stick mode with carbon atoms colored in gray). These arginine and lysine residues generate an electric field that polarizes the thiophosphate moiety, thereby contributing to the 1000-fold tighter binding of the PS2-modified RNA to thrombin relative to the parent aptamer.

Synthesis of the (S)-GNA thymine phosphoramidite from (S)-glycidyl 4,4'-dimethoxytrityl ether. (a) Thymine, NaH, DMF; (b) ClP(NiPr2)(OCE), (iPr2)2NEt. T = thymine. Adapted from [99].
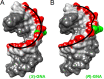
Surface models of the crystal structures of RNA dodecamers with single (A) (S)-GNA-T (PDB ID 5V1L) [54] and (B) (R)-GNA-T (PDB ID 5V1K) [54] nucleotides per strand. The presence of the (R)-GNA isomer introduces a kink in the backbone and causes local disruption of base stacking, in-line with a significantly reduced Tm relative to the (S)-GNA isomer.
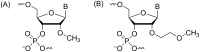
Structures of 2'-O-alkyl modifications. (A) 2'-O-methoxy RNA (2'-OMe RNA), (B) 2'-O-(2-methoxyethyl) RNA (2'-O-MOE RNA).
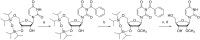
Synthesis of the 2'-OMe uridine from 3',5'-O-(tetraisopropyldisiloxane-1,3-diyl)uridine. (a) Benzoyl chloride, triethylamine; (b) CH3I, Ag2O; (c) dilute NH4OH; (d) 0.5 N HCl. Adapted from [108].
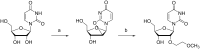
Synthesis of the 2'-O-MOE uridine from uridine. (a) (PhO)2CO, NaHCO3, DMA, 100 °C; (b) Al(OCH2CH2OCH3)3, reflux. Adapted from [117].
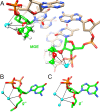
Structure of 2'-O-(2-methoxyethyl)-RNA (MOE-RNA). (A) View into the minor groove of an A-form DNA decamer with single MOE-T nucleotides per strand (PDB ID 411D, highlighted with green carbon atoms) [120]. Water molecules are trapped in a chelate-like manner between the O3', O2' and OC' (outer oxygen of the MOE substituent). (B) and (C) individual nucleotides from a crystal structure of an MOE-RNA dodecamer duplex (PDB ID 469D) [58]. Of the 24 MOE substituents, 22 adopt a gauche conformation, either g+ or g−, whereby both trap a water molecule that can be linked to the 3'-phosphate via a water bridge.
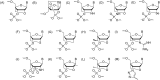
Structures of locked nucleic acids (LNA)/bridged nucleic acids (BNA) modifications. (A) LNA/BNA, (B) α-ʟ-LNA, (C) C2'-amino-LNA, (D) 3'-amino-2',4'-LNA, (E) seleno-LNA, (F) thio-LNA, (G) carba-LNA, (H) S-constrained ethyl (cEt) nucleic acid, (I) 2'-N-guanidino,4'-C-ethylene nucleic acid (GENA), (J) sulfonamide-bridged nucleic acid (suNA), (K) 2'-Me-LNA, (L) 6'-S-Me-2'-O,4'-C-ethylene-bridged nucleic acid (6'-S-Me-ENA), (M) triazole linked LNA.
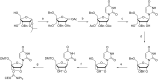
Synthesis of the uridine LNA phosphoramidite. (a) i) NaH, BnBr, DMF, ii) acetic anhydride, pyridine, iii) 80% AcOH, iv) acetic anhydride, pyridine; (b) uracil, N,O-bis(trimethylsilyl)acetamide, TMS-triflate, acetonitrile; (c) NaOCH3, methanol; (d) i) p-toluenesulphonyl chloride, pyridine, ii) NaH, DMF; (e) H2, Pd(OH)2/C, ethanol; (f) DMTCI, pyridine; (g) ClP(NiPr2)(OCE), (iPr2)2NEt, dichloromethane. Bn = benzyl, Ac = acetyl. Adapted from [128].

Synthesis of the 2'-fluoroarabinothymidine. (a) 30% HBr in acetic acid; (b) 2,4-bis-O-(trimethylsilyl)thymine, carbon tetrachloride; (c) NH4OH, methanol. Bz = benzoyl. Adapted from [177].
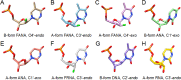
Sugar puckers of arabinose (ANA) and arabinofluoro (FANA) nucleic acids compared with the puckers of the fluoro-ribonucleic acid analog (FRNA) as well as DNA and RNA. (A) FANA-T in B-form DNA (PDB ID 388D) [189]. (B) FANA-T in A-form DNA (PDB ID 2FIL, duplex 1) [190]. (C) FANA-T in A-form DNA (PDB 2FIL, duplex 2) [190]. (D) ANA-U in B-form DNA (PDB ID 2FII) [190]. (E) ANA-U in A-form DNA (PDB ID 2FIJ) [190]. (F) FRNA-U in A-form RNA (PDB ID 3P4A) [62]. (G) B-form DNA (PDB ID 388D) [189]. (H) A-form RNA (PDB ID 5DEK) [199].
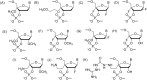
Structures of C4'-modified nucleic acids. (A) 4'-methoxy, (B) 4'-(2-methoxyethoxy), (C) 2',4'-difluoro (2',4'-diF) RNA, (D) 2',4'-difluoro (2',4'-diF) ANA, (E) 2',4'-dimethoxy RNA, (F) 2'-methoxy,4'-fluoro RNA, (G) 2'-fluoro,4'-methoxy ANA, (H) 4'-fluoro RNA, (I) 4'-C-aminoalkyl-2'-O-methyl, (J) 4'-C-aminoalkyl-2'-fluoro, (K) 4'-C-guanidinocarbohydrazidomethyl.
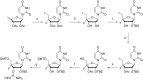
Synthesis of the 4'-F-rU phosphoramidite. (a) AgF, I2, dichloromethane, tetrahydrofuran; (b) NH3, methanol; (c) TBS-Cl, AgNO3, pyridine, tetrahydrofuran; (d) acetic anhydride, dimethylaminopyridine, pyridine; (e) tetrabutylammonium hydroxide, trifluoroacetic acid, m-chloroperoxybenzoic acid; (f) (i) NH3, methanol (ii) DMTCI, pyridine; (g) ClP(NiPr2)(OCE), 1-methylimidazole, (iPr2)2NEt, dichloromethane. TBS = tert-butyldimethylsilyl. Adapted from [211].
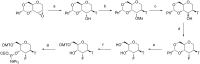
Synthesis of the thymine FHNA phosphoramidite. (a) thymine, 1,8-diazabicyclo[5.4.0]undec-7-ene, acetonitrile; (b) methanesulfonyl chloride, pyridine; (c) aq NaOH, 1,4-dioxane; (d) nonafluorobutanesulfonyl fluoride, 1,8-diazabicyclo[5.4.0]undec-7-ene, tetrahydrofuran; (e) H2, Pd(OH)2/C, methanol; (f) DMTCI, pyridine; (g) P(NiPr2)2(OCE), 1H-tetrazole, NMI, DMF. Ms = methanesulfonyl, Ph = phenyl, T = thymine. Adapted from [221].
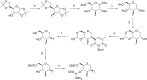
Synthesis of the thymine Ara-FHNA phosphoramidite. (a) i) trifluoromethanesulfonic anhydride, pyridine, ii) CsF, tert-butanol; (b) i) Amberlite IR-120-H, 1,4-dioxane, water, ii) acetic anhydride, pyridine; (c) i) 33% HBr in acetic acid, dichloromethane, ii) tributyltin hydride, 2,2′-azobis(2-methylpropionitrile), toluene; (d) i) K2CO3, methanol, ii) benzaldehyde dimethyl acetal, p-toluenesulfonic acid, DMF; (e) i) trifluoromethanesulfonic anhydride, dichloromethane, pyridine, ii) N3-benzyloxymethylthymine, 1,8-diazabicyclo[5.4.0]undec-7-ene, dimethyl sulfoxide; (f) H2, Pd(OH)2/C, methanol; (g) DMTCI, pyridine; (h) P(NiPr2)2(OCE), 1H-tetrazole, NMI, DMF. Bom = benzyloxymethyl. Adapted from [221].
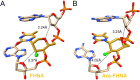
Crystal structures of (A) FHNA and (B) Ara-FHNA in modified A-form DNA decamers (PDB IDs 3Q61 and 3SD8, respectively) [221]. Unlike the trans-diaxial orientation of the fluorine in FHNA, the equatorial orientation of fluorine in Ara-FHNA pushes away the 3'-adjacent nucleotide (dashed lines) and causes local unstacking of bases.
Similar articles
-
Re-Engineering RNA Molecules into Therapeutic Agents.
Egli M, Manoharan M. Egli M, et al. Acc Chem Res. 2019 Apr 16;52(4):1036-1047. doi: 10.1021/acs.accounts.8b00650. Epub 2019 Mar 26. Acc Chem Res. 2019. PMID: 30912917
-
Egli M, Pallan PS. Egli M, et al. Annu Rev Biophys Biomol Struct. 2007;36:281-305. doi: 10.1146/annurev.biophys.36.040306.132556. Annu Rev Biophys Biomol Struct. 2007. PMID: 17288535 Review.
-
2'-Modified oligonucleotides for antisense therapeutics.
Prakash TP, Bhat B. Prakash TP, et al. Curr Top Med Chem. 2007;7(7):641-9. doi: 10.2174/156802607780487713. Curr Top Med Chem. 2007. PMID: 17430205 Review.
-
Egli M, Minasov G, Tereshko V, Pallan PS, Teplova M, Inamati GB, Lesnik EA, Owens SR, Ross BS, Prakash TP, Manoharan M. Egli M, et al. Biochemistry. 2005 Jun 28;44(25):9045-57. doi: 10.1021/bi050574m. Biochemistry. 2005. PMID: 15966728
-
The Medicinal Chemistry of Artificial Nucleic Acids and Therapeutic Oligonucleotides.
Bege M, Borbás A. Bege M, et al. Pharmaceuticals (Basel). 2022 Jul 22;15(8):909. doi: 10.3390/ph15080909. Pharmaceuticals (Basel). 2022. PMID: 35893733 Free PMC article. Review.
Cited by
-
Synthesis and Properties of RNA Modified with Cationic Amine Internucleoside Linkage.
Pal C, Richter M, Rozners E. Pal C, et al. ACS Chem Biol. 2024 Feb 16;19(2):249-253. doi: 10.1021/acschembio.3c00784. Epub 2024 Feb 5. ACS Chem Biol. 2024. PMID: 38314716
-
Hyjek-Składanowska M, Anderson BA, Mykhaylyk V, Orr C, Wagner A, Poznański JT, Skowronek K, Seth P, Nowotny M. Hyjek-Składanowska M, et al. Nucleic Acids Res. 2023 Feb 22;51(3):1409-1423. doi: 10.1093/nar/gkac774. Nucleic Acids Res. 2023. PMID: 36124719 Free PMC article.
-
Aralov AV, Gubina N, Cabrero C, Tsvetkov VB, Turaev AV, Fedeles BI, Croy RG, Isaakova EA, Melnik D, Dukova S, Ryazantsev DY, Khrulev AA, Varizhuk AM, González C, Zatsepin TS, Essigmann JM. Aralov AV, et al. Nucleic Acids Res. 2022 Apr 8;50(6):3056-3069. doi: 10.1093/nar/gkac148. Nucleic Acids Res. 2022. PMID: 35234900 Free PMC article.
-
Fluorobenzene Nucleobase Analogues for Triplex-Forming Peptide Nucleic Acids.
Kumar V, Rozners E. Kumar V, et al. Chembiochem. 2022 Feb 4;23(3):e202100560. doi: 10.1002/cbic.202100560. Epub 2021 Dec 20. Chembiochem. 2022. PMID: 34889020 Free PMC article.
-
Artificial genetic polymers against human pathologies.
Ivanov GS, Tribulovich VG, Pestov NB, David TI, Amoah AS, Korneenko TV, Barlev NA. Ivanov GS, et al. Biol Direct. 2022 Dec 6;17(1):39. doi: 10.1186/s13062-022-00353-7. Biol Direct. 2022. PMID: 36474260 Free PMC article. Review.
References
Publication types
LinkOut - more resources
Full Text Sources
Other Literature Sources
Miscellaneous