Recent advances in drug delivery applications of cubosomes, hexosomes, and solid lipid nanoparticles - PubMed
Review
Recent advances in drug delivery applications of cubosomes, hexosomes, and solid lipid nanoparticles
Anan Yaghmur et al. Acta Pharm Sin B. 2021 Apr.
Abstract
The use of lipid nanocarriers for drug delivery applications is an active research area, and a great interest has particularly been shown in the past two decades. Among different lipid nanocarriers, ISAsomes (Internally self-assembled somes or particles), including cubosomes and hexosomes, and solid lipid nanoparticles (SLNs) have unique structural features, making them attractive as nanocarriers for drug delivery. In this contribution, we focus exclusively on recent advances in formation and characterization of ISAsomes, mainly cubosomes and hexosomes, and their use as versatile nanocarriers for different drug delivery applications. Additionally, the advantages of SLNs and their application in oral and pulmonary drug delivery are discussed with focus on the biological fates of these lipid nanocarriers in vivo. Despite the demonstrated advantages in in vitro and in vivo evaluations including preclinical studies, further investigations on improved understanding of the interactions of these nanoparticles with biological fluids and tissues of the target sites is necessary for efficient designing of drug nanocarriers and exploring potential clinical applications.
Keywords: Biological fate; Cubosomes; Drug delivery; Hexosomes; Inverse non-lamellar liquid crystalline phases; Nano-self-assemblies; Solid crystalline phases; Solid lipid nanoparticles.
© 2021 Chinese Pharmaceutical Association and Institute of Materia Medica, Chinese Academy of Medical Sciences. Production and hosting by Elsevier B.V.
Conflict of interest statement
The authors have no conflicts of interest to declare.
Figures
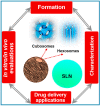
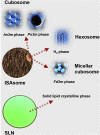
Schematic illustration of representative nano-lamellar nano-self-assemblies with unique structural features and solid lipid nanoparticles (SLNs). These nanoparticles are attractive for use as nanocarriers for drug delivery and bio-imaging applications.
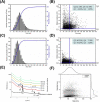
Size and structural characterization of ISAsomes. NTA results showing size distribution profiles (panels A and C), and relative light scattering intensities (panels B and D), before (panels A and B) and after (panels C and D) incubation of F127-stabilized PHYT nanodispersion with human plasma. In the presence of plasma, comparison of size distribution profiles indicated that plasma-mediated loss of some relatively larger nanoparticles (≥150 nm) with concomitant decrease in intensity. Characterization of F127-stabilized PHYT/oleic acid (OA) hexosomes by synchrotron SAXS (E) and NTA (F). In panel E, black colored SAXS pattern indicating the formation of unlabelled hexosomes at 37 °C, and effect of swelling at different time points on incubation of the nanodispersion with rat plasma. (F) Relative light scattering intensity for unlabelled (black) and (99mTc)-labelled hexosomes (grey). Panels A–D were taken with permission from Ref. ; whereas panels E and F were taken with permission from Ref. .
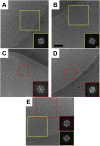
Morphological characterization of ISAsomes. Cryo-TEM images of selected F127-stabilized nanodispersions after vitrification at 37 °C. (A) unlabeled PEGylated PHYT nanodispersion; (B) labelled PEGylated PHYT nanodispersion with 99mTc-HMPAO (technetium-99 m labelling by using the chelating agent hexamethylpropyleneamine oxime, HMPAO); (C) unlabeled PHYT/OA hexosomes; (D) unlabeled PHYT nanodispersion; (E) unlabeled PHYT/1, 2-distearoyl-sn-glycero-3-phosphoethanolamine (DSPE) nanoparticles. Red insets reveal the fast Fourier transformation (FFT) analysis of the observed hexosomes displaying internal H2 phase, whereas yellow insets display FFT analysis of cubosomes with internal inverse bicontinuous cubic Pn3m phase. Scale bar: 100 nm. This figure was adapted with permission from Ref. .
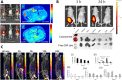
(A) NIRF imaging of whole body of mice after tail vein injections of cubosomes (cub) and hexosomes (hex): a) immediately after injection, and b) at 120 min of post injection. In c and d, enhanced in vivo MRI images (marked by dotted lines) of spleen and liver at 30 min post-injection of NIRF-MRI cubosomes are shown. Adapted with permission from Ref. . (B) In the left: whole body imaging of A431 tumor-bearing mice at 3 and 24 h of i.p. post-administration of paclitaxel-containing cubosomes. In the right: ex vivo imaging of organs at 24 h of post-injection. Adapted with permission from Ref. . (C) Whole body SPECT/CT imaging and biodistribution of 99mTc-labeled-hexosomes on footpad s.c. injection. Thick yellow arrows indicate accumulated formulation at the footpad site. Single yellow solid arrow = popliteal node; double yellow solid arrow = iliac node; dashed arrow = inguinal node; K = kidney; B = bladder. Biodistribution: radiotracer contents in the whole footpad, regional lymph nodes, blood pool, liver, and kidneys at different post-injection times, as determined by γ-counting, are shown in panels (i)‒(v). Adapted with permission from Ref. .
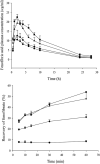
(A) Plasma concentration–time profile following oral administration of 100 nm LMPs (), 400 nm LMPs (
), microparticles (
) and control (
) in male Wistar rats (fenofibrate dosed at 12.5 mg/animal, n = 6, mean ± SEM). (B) Recovery of fenofibrate (%) in the aqueous phase (mean ± SEM) during in vitro lipolysis of 100 nm SLN (
), 400 nm SLN (
), microparticles (
) and control (
) (n = 3 except 100 nm, 60 min is n = 1 (SEM not shown) and 400 nm, 60 min is n = 2). Modified with permission from Ref. .
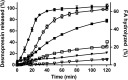
Release of desmopressin during in vitro lipolysis (closed symbols) and amount of hydrolyzed fatty acids (open symbols) from triglyceride (TG) particles. , TG14 particles;
, TG16 particles;
, TG18 particles. Data are expressed as mean ± SD (n = 3). Reproduced with permission from Ref. .
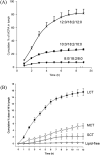
Lymphatic transport of lipids and halofantrine solubilised in lipids. (A) Cumulative lymphatic transport of fatty acids after intragastric administration of structured TG 8:0/18:2/8:0, 10:0/18:2/10:0, 12:0/18:2/12:0; (B) cumulative lymphatic transport of halofantrine after oral administration in different TG solutions. Reproduced with permission from Ref. .
Similar articles
-
Cubosomes and Hexosomes as Novel Nanocarriers for Bioactive Compounds.
Tan C, Hosseini SF, Jafari SM. Tan C, et al. J Agric Food Chem. 2022 Feb 9;70(5):1423-1437. doi: 10.1021/acs.jafc.1c06747. Epub 2022 Jan 28. J Agric Food Chem. 2022. PMID: 35089018 Review.
-
Cubosomes and hexosomes as versatile platforms for drug delivery.
Azmi ID, Moghimi SM, Yaghmur A. Azmi ID, et al. Ther Deliv. 2015;6(12):1347-64. doi: 10.4155/tde.15.81. Ther Deliv. 2015. PMID: 26652281 Review.
-
Inverse ISAsomes in Bio-Compatible Oils-Exploring Formulations in Squalane, Triolein and Olive Oil.
Trummer F, Glatter O, Chemelli A. Trummer F, et al. Nanomaterials (Basel). 2022 Mar 29;12(7):1133. doi: 10.3390/nano12071133. Nanomaterials (Basel). 2022. PMID: 35407249 Free PMC article.
-
Zhai J, Fong C, Tran N, Drummond CJ. Zhai J, et al. ACS Nano. 2019 Jun 25;13(6):6178-6206. doi: 10.1021/acsnano.8b07961. Epub 2019 May 22. ACS Nano. 2019. PMID: 31082192 Review.
-
Temperature triggering of kinetically trapped self-assemblies in citrem-phospholipid nanoparticles.
Prajapati R, Salentinig S, Yaghmur A. Prajapati R, et al. Chem Phys Lipids. 2018 Nov;216:30-38. doi: 10.1016/j.chemphyslip.2018.09.003. Epub 2018 Sep 14. Chem Phys Lipids. 2018. PMID: 30222973
Cited by
-
Wu Y, Angelova A. Wu Y, et al. Nanomaterials (Basel). 2023 Nov 23;13(23):3004. doi: 10.3390/nano13233004. Nanomaterials (Basel). 2023. PMID: 38063700 Free PMC article. Review.
-
Nazaruk E, Gajda E, Ziędalska I, Godlewska M, Gawel D. Nazaruk E, et al. ACS Omega. 2024 Sep 2;9(37):38936-38945. doi: 10.1021/acsomega.4c05291. eCollection 2024 Sep 17. ACS Omega. 2024. PMID: 39310207 Free PMC article.
-
Functional nano-systems for transdermal drug delivery and skin therapy.
Liu L, Zhao W, Ma Q, Gao Y, Wang W, Zhang X, Dong Y, Zhang T, Liang Y, Han S, Cao J, Wang X, Sun W, Ma H, Sun Y. Liu L, et al. Nanoscale Adv. 2023 Feb 24;5(6):1527-1558. doi: 10.1039/d2na00530a. eCollection 2023 Mar 14. Nanoscale Adv. 2023. PMID: 36926556 Free PMC article. Review.
-
Farag DBE, Yousry C, Al-Mahallawi AM, El-Askary HI, Meselhy MR, AbuBakr N. Farag DBE, et al. Drug Deliv. 2022 Dec;29(1):62-74. doi: 10.1080/10717544.2021.2018522. Drug Deliv. 2022. PMID: 34964423 Free PMC article.
-
Lipid carriers for mRNA delivery.
Zhang W, Jiang Y, He Y, Boucetta H, Wu J, Chen Z, He W. Zhang W, et al. Acta Pharm Sin B. 2023 Oct;13(10):4105-4126. doi: 10.1016/j.apsb.2022.11.026. Epub 2022 Nov 30. Acta Pharm Sin B. 2023. PMID: 37799378 Free PMC article. Review.
References
-
- Couvreur P., Vauthier C. Nanotechnology: intelligent design to treat complex disease. Pharm Res (N Y) 2006;23:1417–1450. - PubMed
-
- Moghimi S.M., Hunter A.C., Murray J.C. Nanomedicine: current status and future prospects. Faseb J. 2005;19:311–330. - PubMed
-
- Wibroe P.P., Ahmadvand D., Oghabian M.A., Yaghmur A., Moghimi S.M. An integrated assessment of morphology, size, and complement activation of the PEGylated liposomal doxorubicin products Doxil®, Caelyx®, DOXOrubicin, and SinaDoxosome. J Control Release. 2016;221:1–8. - PubMed
-
- Bor G., Mat Azmi I.D., Yaghmur A. Nanomedicines for cancer therapy: current status, challenges and future prospects. Ther Deliv. 2019;10:113–132. - PubMed
Publication types
LinkOut - more resources
Full Text Sources
Other Literature Sources
Miscellaneous