The stromal vascular fraction mitigates radiation-induced gastrointestinal syndrome in mice - PubMed
- ️Fri Jan 01 2021
The stromal vascular fraction mitigates radiation-induced gastrointestinal syndrome in mice
Lydia Bensemmane et al. Stem Cell Res Ther. 2021.
Abstract
Background: The intestine is particularly sensitive to moderate-high radiation dose and the development of gastrointestinal syndrome (GIS) leads to the rapid loss of intestinal mucosal integrity, resulting in bacterial infiltration, sepsis that comprise patient survival. There is an urgent need for effective and rapid therapeutic countermeasures. The stromal vascular fraction (SVF) derived from adipose tissue is an easily accessible source of cells with angiogenic, anti-inflammatory and regenerative properties. We studied the therapeutic impact of SVF and its action on the intestinal stem cell compartment.
Methods: Mice exposed to the abdominal radiation (18 Gy) received a single intravenous injection of stromal vascular fraction (SVF) (2.5 × 106 cells), obtained by enzymatic digestion of inguinal fat tissue, on the day of irradiation. Mortality was evaluated as well as intestinal regeneration by histological analyses and absorption function.
Results: The SVF treatment limited the weight loss of the mice and inhibited the intestinal permeability and mortality after abdominal irradiation. Histological analyses showed that SVF treatment stimulated the regeneration of the epithelium by promoting numerous enlarged hyperproliferative zones. SVF restored CD24+/lysozyme- and Paneth cell populations in the ISC compartment with the presence of Paneth Ki67+ cells. SVF has an anti-inflammatory effect by repressing pro-inflammatory cytokines, increasing M2 macrophages in the ileum and anti-inflammatory monocyte subtypes CD11b+Ly6clowCX3CR1high in the spleen.
Conclusions: Through the pleiotropic effects that contribute to limiting radiation-induced lethality, SVF opens up attractive prospects for the treatment of emergency GIS.
Keywords: Intestine; Irradiation; Regeneration; Stromal vascular fraction.
Conflict of interest statement
The authors declare that they have no competing interests.
Figures
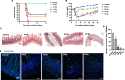
Identification of irradiation dose inducing GIS. a Kaplan-Meier survival analysis of 16, 18, 20 and 22 Gy abdominal irradiated mice (n = 10 per group). b Weight loss changes for 30 days of mice exposed to 16, 18, 20 and 22 Gy abdominal irradiation (n = 10 per group). c Response of the small intestine to 16, 18, 20 and 22 Gy abdominal irradiation at day 3.5. Representative HES staining of the ileum showing structural modification. Scale bar 100 μm. d Irradiation dose-dependent regenerative crypts. The plots illustrate the number of surviving regenerative crypts present by unit length in a small intestine 3.5 days post-irradiation. The data are represented by mean ± SEM (n = 4–5), p values were calculated by one-way or two-way analyses (for weight loss changes) of variance (ANOVA) with Bonferroni correction; *p < 0.01; **p < 0.001 compared with the control group. e Tunel staining. Representative images of ileal sections at 3.5 days post-irradiation demonstrating an increase of Tunel-positive cells at the crypt base with the escalating dose from 16 to 22 Gy. Tunel-positive cells were stained green and the nucleus was stained with DAPI at day 3.5 post-irradiation. Scale bar 50 μm
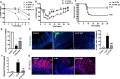
SVF treatment mitigating GIS. a Cell concentration-dependent effect of SVF on weight loss changes at 7 days of mice exposed to 18 Gy abdominal irradiation. b Weight loss changes for 30 days (n = 8) and c Kaplan-Meier survival analysis of 18 Gy abdominal exposed mice receiving SVF (2.5 × 106 cells) treatment, or not receiving treatment. The data are represented by mean ± SEM; p values were calculated by ANOVA with Bonferroni correction; *p < 0.05; **p < 0.01; ***p < 0.001 compared with the irradiated group. d Number of regenerative crypts at day 3.5 after 18 Gy abdominal irradiation with or without SVF (2.5 × 106 cells) treatment. e Representative immunostaining of Tunel and the number of Tunel+ cells in the ileal sections from the control in irradiated and irradiated SVF-treated mice 7 days post-irradiation. f Intestinal permeability checked by FITC/dextran assay in non-irradiated mice and 7 days after abdominal irradiation with or without SVF treatment. g Representative immunostaining of Zonulin-1 (ZO-1) in the ileal sections from the control in irradiated and irradiated SVF-treated mice 7 days post-irradiation. Scale bar 50 μm. The data are represented by mean ± SEM (n = 8). p values were calculated by ANOVA with Bonferroni correction; ***p < 0.001 compared with the control mice; ###p < 0.001 compared with the irradiated mice
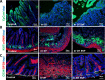
Ileal regenerative response induced by SVF treatment. Sections of ileum from mice 7 days after 18 Gy abdominal irradiation, with or without SVF treatment were stained with a EpCam (green) and b Ki67 (green). Note the hypertrophic (green) crypts under SVF treatment. c Co-staining of gp38 (red) and CD34 (green) identifying pericryptal mesenchymal cells. Double-positive gp38+CD34+ appeared in orange/yellow at the base of crypts. Dapi stained nuclei. Scale bars 50 μm
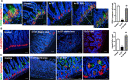
Effect of SVF on crypt IECS. a IHC staining showing the expression pattern of CD24 (green) and lysozyme (red) in the ileum. The cross section shows the co-staining of CD24 and lysozyme in the control and after SVF treatment. The histogram shows the restoration of the CD24 population after SVF treatment in crypts. b Lysozyme staining and histogram of the Paneth cell number per crypts. c IHC co-stained with lysozyme (red) and goblet cell marker Muc2 (green) to identify immature Paneth progenitor cells. Dapi stained nuclei. Scale bars 50 μm. The data are represented by mean ± SEM (n = 4–5). p values were calculated by ANOVA with Bonferroni correction, *p < 0.01; **p < 0.001 compared with the control group; ##p < 0.001 compared with the irradiated group
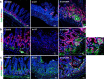
Crypt IECS proliferation induced by SVF treatment. a IHC co-staining CD24 (green) and Ki67 (red) in the ileum showing the hyperproliferative area after SVF treatment. b IHC co-staining lysozyme (green) and Ki67 (red) in the ileum. c IHC co-staining HSP60 (green) and Ki67 (red) in the ileum. Dapi stained nuclei
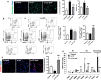
SVF promoting anti-inflammatory monocyte/macrophage subsets in the spleen. a Phalloidine staining (green) of splenic monocytes from the control and at day 7 post-irradiation with or without SVF treatment. Dapi stained nuclei. Scale bar 20 μm. The bar graphs are represented by the mean ± SEM of monocytes phalloidine+ and the monocyte diameters. b Representative FACS plots and frequencies of CD11b+Ly6ChighCCR2high and CD11b+Ly6ClowCx3cr1high among live cells from the control and at day 7 post-irradiation with or without SVF treatment. c Cx3cr1staining (red) of splenic monocytes. The bar graphs are represented by the mean ± SEM of Cx3CR1+ monocytes. Dapi stained nuclei. Scale bar 20 μm. d Gene expression from splenic monocytes differentiated in different culture conditions from the control and at day 7 post-irradiation with or without SVF treatment. Macrophages were generated from splenic monocytes in the presence of M-CSF. Real-time PCR in an unstimulated condition, a M1 condition after stimulation with LPS and IFN-γ and a M2a condition after stimulation with IL-4. The data are expressed relative to control and normalised to GAPDH. All results are expressed as means ±SEM (n = 8). p values were calculated by ANOVA with Bonferroni correction, *p < 0.05; **p < 0.01; ***p < 0.001 compared with the control group; #p < 0.05; ##p < 0.01; ###p < 0.001 compared with the irradiated group
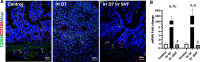
SVF facilitated induction of M2 macrophages in the ileum and reduced radiation-induced inflammatory response. a IHC co-staining CD68 (green) and CD206 (red) in the ileum showing an increase of the co-staining CD68/CD206 related to M2 macrophage after SVF treatment. Dapi stained nuclei. b Real-time PCR analysis of IL-1β and IL-6. The results are expressed as means ±SEM (n = 8). p values were calculated by analysis of variance with Bonferroni correction; *p < 0.001 compared with the control mice; #p < 0.001 compared with the irradiated mice
Similar articles
-
Bensemmane L, Milliat F, Treton X, Linard C. Bensemmane L, et al. Stem Cell Res Ther. 2023 Nov 13;14(1):325. doi: 10.1186/s13287-023-03562-7. Stem Cell Res Ther. 2023. PMID: 37953266 Free PMC article.
-
Saha S, Bhanja P, Kabarriti R, Liu L, Alfieri AA, Guha C. Saha S, et al. PLoS One. 2011;6(9):e24072. doi: 10.1371/journal.pone.0024072. Epub 2011 Sep 15. PLoS One. 2011. PMID: 21935373 Free PMC article.
-
Dong Z, Fu R, Liu L, Lu F. Dong Z, et al. Cell Biol Int. 2013 Aug;37(8):855-9. doi: 10.1002/cbin.10099. Epub 2013 Apr 18. Cell Biol Int. 2013. PMID: 23526646
-
Adipose-Derived Stromal Vascular Fraction Cells: Update on Clinical Utility and Efficacy.
Han S, Sun HM, Hwang KC, Kim SW. Han S, et al. Crit Rev Eukaryot Gene Expr. 2015;25(2):145-52. doi: 10.1615/critreveukaryotgeneexpr.2015013057. Crit Rev Eukaryot Gene Expr. 2015. PMID: 26080608 Review.
-
Stromal vascular fraction: A regenerative reality? Part 2: Mechanisms of regenerative action.
Guo J, Nguyen A, Banyard DA, Fadavi D, Toranto JD, Wirth GA, Paydar KZ, Evans GR, Widgerow AD. Guo J, et al. J Plast Reconstr Aesthet Surg. 2016 Feb;69(2):180-8. doi: 10.1016/j.bjps.2015.10.014. Epub 2015 Oct 24. J Plast Reconstr Aesthet Surg. 2016. PMID: 26546112 Review.
Cited by
-
Bensemmane L, Milliat F, Treton X, Linard C. Bensemmane L, et al. Stem Cell Res Ther. 2023 Nov 13;14(1):325. doi: 10.1186/s13287-023-03562-7. Stem Cell Res Ther. 2023. PMID: 37953266 Free PMC article.
-
Wu L, Chen L, Li H, Wang Y, Xu K, Chen W, Zhang A, Wang Y, Shi C. Wu L, et al. Burns Trauma. 2024 Mar 4;12:tkad045. doi: 10.1093/burnst/tkad045. eCollection 2024. Burns Trauma. 2024. PMID: 38444637 Free PMC article.
-
A Fiber-Rich Diet and Radiation-Induced Injury in the Murine Intestinal Mucosa.
Malipatlolla DK, Devarakonda S, Patel P, Sjöberg F, Rascón A, Grandér R, Skokic V, Kalm M, Danial J, Mehdin E, Warholm M, Norling H, Stringer A, Johansson MEV, Nyman M, Steineck G, Bull C. Malipatlolla DK, et al. Int J Mol Sci. 2021 Dec 31;23(1):439. doi: 10.3390/ijms23010439. Int J Mol Sci. 2021. PMID: 35008864 Free PMC article.
-
Dushime H, Moreno SG, Linard C, Adrait A, Couté Y, Peltzer J, Messiaen S, Torres C, Bensemmane L, Lewandowski D, Romeo PH, Petit V, Gault N. Dushime H, et al. Stem Cell Res Ther. 2023 Aug 11;14(1):201. doi: 10.1186/s13287-023-03425-1. Stem Cell Res Ther. 2023. PMID: 37568164 Free PMC article.
-
Horseman T, Rittase WB, Slaven JE, Bradfield DT, Frank AM, Anderson JA, Hays EC, Ott AC, Thomas AE, Huppmann AR, Lee SH, Burmeister DM, Day RM. Horseman T, et al. Int J Mol Sci. 2024 Apr 20;25(8):4535. doi: 10.3390/ijms25084535. Int J Mol Sci. 2024. PMID: 38674120 Free PMC article.
References
-
- Krivokrysenko VI, Shakhov AN, Singh VK, Bone F, Kononov Y, Shyshynova I, Cheney A, Maitra RK, Purmal A, Whitnall MH, Gudkov AV, Feinstein E. Identification of granulocyte colony-stimulating factor and interleukin-6 as candidate biomarkers of CBLB502 efficacy as a medical radiation countermeasure. J Pharmacol Exp Ther. 2012;343(2):497–508. doi: 10.1124/jpet.112.196071. - DOI - PMC - PubMed
Publication types
MeSH terms
LinkOut - more resources
Full Text Sources
Other Literature Sources
Research Materials