Functional Characterization of Hexacorallia Phagocytic Cells - PubMed
- ️Fri Jan 01 2021
Functional Characterization of Hexacorallia Phagocytic Cells
Grace A Snyder et al. Front Immunol. 2021.
Abstract
Phagocytosis is the cellular defense mechanism used to eliminate antigens derived from dysregulated or damaged cells, and microbial pathogens. Phagocytosis is therefore a pillar of innate immunity, whereby foreign particles are engulfed and degraded in lysolitic vesicles. In hexacorallians, phagocytic mechanisms are poorly understood, though putative anthozoan phagocytic cells (amoebocytes) have been identified histologically. We identify and characterize phagocytes from the coral Pocillopora damicornis and the sea anemone Nematostella vectensis. Using fluorescence-activated cell sorting and microscopy, we show that distinct populations of phagocytic cells engulf bacteria, fungal antigens, and beads. In addition to pathogenic antigens, we show that phagocytic cells engulf self, damaged cells. We show that target antigens localize to low pH phagolysosomes, and that degradation is occurring within them. Inhibiting actin filament rearrangement interferes with efficient particle phagocytosis but does not affect small molecule pinocytosis. We also demonstrate that cellular markers for lysolitic vesicles and reactive oxygen species (ROS) correlate with hexacorallian phagocytes. These results establish a foundation for improving our understanding of hexacorallian immune cell biology.
Keywords: FACS; Hexacorallia; Sea anemone; coral immunity; coral reefs; flow cytometry; innate immunity; phagocytosis.
Copyright © 2021 Snyder, Eliachar, Connelly, Talice, Hadad, Gershoni-Yahalom, Browne, Palmer, Rosental and Traylor-Knowles.
Conflict of interest statement
The authors declare that the research was conducted in the absence of any commercial or financial relationships that could be construed as a potential conflict of interest.
Figures
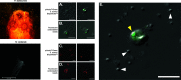
FACS-isolated cells of Pocillopora damicornis and Nematostella vectensis positive for phagocytosis. (A–E) Images of sorted cells positive for S. aureus and bead assays. (A) Images of sorted cells from P. damicornis challenged with inactive pHrodo™ S. aureus particles, with the center host cells having internalized, pH-activated bacteria. (B) Images of sorted cells from P. damicornis challenged with fluorescent carboxylated beads, with the center host cells having internalized beads. (C) Images of sorted cells from N. vectensis challenged with inactive pHrodo™ S. aureus particles, with the CellTrace-stained animal cells having internalized, pH-activated bacteria. (D) Images of sorted cells from N. vectensis challenged with fluorescent carboxylated beads, with the CellTrace-stained cells having internalized beads. (E) Enlarged version of right image in (A), a P. damicornis cell challenged with S. aureus. Yellow arrow shows an internalized, pH-activated S. aureus particle that fluoresces bright green at a low pH. White arrows indicate the cocci bacterial particles. S. aureus particles are fluorescent after the fusion of the bacteria with the lysolitic vesicle, which leads to lowered pH. This is not seen in the free bacteria that are not internalized by the cells and can be seen only with DIC. All P. damicornis pictures were taken on a ZEISS Axio Imager.Z2 microscope with a magnification of 40X using a combination of DIC and 470nm LED with ZEISS 38HE filter set. All N. vectensis pictures were taken on ZEISS LSM900 confocal microscope with a magnification of 40X using a combination of PMT, green and far-red filters. White scale bars represent 10 μm.
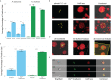
Phagocytosis in P. damicornis and N. vectensis of different antigens, and their fusion with low pH vesicles and protein degradation. (A) Percentage of cells in P. damicornis (blue bars) and N. vectensis (green bars) that engulfed pHrodo™ E. coli (left) and pHrodo™ zymosan (right) particles. Each test treatment is significantly different from the respective control treatment. (B) Images of isolated N. vectensis cells challenged with pHrodo-red™ E. coli particles, taken by confocal microscopy. Left: internalized, pH-activated E. coli particles that fluoresce brightly in acidic environments. Middle: N. vectensis stained with CellTrace. Right: Overlays of left and middle panels in confocal microscopy PMT. E. coli particles fluoresce after the fusion with low pH lysolitic vesicles. Scale bar for upper panels 2 μm, and 5 μm for lower panels. (C) Percentage of cells in P. damicornis (blue bars) and N. vectensis (green bars) that engulfed DQ™ ovalbumin, a particle that only fluoresces bright green when hydrolysis by proteases due to the quenching of the reagent. The number of cells engulfing the ovalbumin is significantly higher than that of the control treatment. (D) 3D analysis of a N. vectensis cell stained with CellTrace and has been exposed to DQ™ ovalbumin. Left: 2D overlay of confocal microscopy and PMT, bar-2 μm. Middle: 3D surface analysis of the same N. vectensis cell featured in the left panel and shows the absence of DQ™ ovalbumin from the surface of the cell. Right: 3D cross-sectional analysis of the same N. vectensis cell featured in the left and middle panels and shows fluorescing DQ™ ovalbumin particles within the cell. The yellow plane depicts the cross section done in the Z-axis (right panel), and surface analysis of the parts below the plane to show what is inside the cells. Grid scales are 3 μm. (E) Inserts of ImageStream analysis of N. vectensis cell stained with CellTrace (red) and has been exposed to DQ™ ovalbumin (green upon protein hydrolysis), scale bar 10 μm. P-value marks: **p < 0.01, ***p < 0.001.
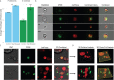
Phagocytosis in P. damicornis and N. vectensis of damaged cells. (A) Percentage of cells in P. damicornis (blue bars) and N. vectensis (green bars) that engulfed cells. Cells from different animals were differentially labeled (CellTrace and CFSE), to detect double positive events that would indicate engulfment of cells. The control group was exposed to cells kept at ambient conditions, while the heat stress group (both labeled with CFSE) was exposed to cells that were kept at 42°C for one hour. Each test treatment is significantly different from the control treatment. (B) Inserts of ImageStream analysis of N. vectensis cells stained with CellTrace (red) and cells stained with CFSE (green) that were heat stressed. The images are examples of the gated double positive population for validation of cells, or their particles engulfed by the red cells. Scale bar 7 μm. (C) Example of 2D images slides in confocal microscopy of N. vectensis cells stained with CellTrace (red) and cells stained with CFSE (green) that were heat stressed. For validation at least 40 slices were taken on a Z axis to create 3D surface analysis (in D). Scale bars 5 μm. (D) Inserts of 3D surface analysis to show the full cells (right panels), of the examples in (C) The yellow plane depicts the cross section done in the Z-axis (right panels), and surface analysis of the parts below the plane to show what is inside the cells. In the center of the image CFSE labeled cells can be seen engulfed by CellTrace labeled cells. Grid scales for the upper panel are 4 μm, and 3 μm for the lower panel. P-value marks: *p < 0.05, ***p < 0.001.
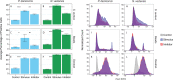
Phagocytosis is a distinct process from pinocytosis and is reliant on actin filament movement. (A, C, E) show the percentage of P. damicornis cells positive for each assay while (B, D, F) show N. vectensis. Error bars are representative of the standard error of the mean. (A, B) Cellular uptake of inactive S. aureus particles is significantly higher in comparison with unexposed controls (p-value in both species < 0.001). The cytochalasin inhibitor resulted in a statistically significant reduction of cellular uptake of inactive S. aureus particles (P. damicornis: 25% reduction, p-value < 0.01; N. vectensis: 42% reduction, p-value < 0.001). (C, D) Similar to the inactive S. aureus particles assay, cellular uptake of green carboxylated beads is significantly higher in the stimulus group compared to an unexposed control (P. damicornis p-value < 0.05; N. vectensis p-value < 0.001). The cytochalasin inhibitor resulted in a reduction of cellular uptake of green carboxylated beads, which was significant in N. vectensis (27% reduction, p-value < 0.01), but only at 90% confidence in P. damicornis (68% reduction, p-value = 0.072). (E, F) 66.5% of cells in P. damicornis and 82% of cells in N. vectensis were detectable after treatment of dextran particles, indicating that particle uptake was ubiquitous. The cytochalasin inhibitor did not cause a statistically significant decrease in dextran uptake, indicating that this process is not facilitated by phagocytosis, but rather pinocytosis. (G–L) FACS data for each assay on a histogram of the single filter (FITC). (G–J) In the phagocytic assays, the inhibitor sample shows a similar histogram profile as the stimulus, with a reduction in cells with high levels of fluorescence. (K, L) The pinocytic assay of dextran exposure shows nearly no difference between the stimulus and inhibitor samples. P-value marks: *p < 0.05, **p < 0.01, ***p < 0.001.
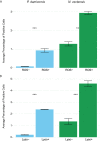
Phagocytic cells are associated with immune cell vesicular markers for ROS (CellROX) and lysosomal vesicles (LysoTracker) in P. damicornis and N. vectensis. For each species, cells were differentially isolated and sorted based on ROS (A) or lysosomal vesicle (B) signals, and then co-incubated with beads. (A) Comparison of low and high levels of ROS in P. damicornis (blue bars) and N. vectensis (green bars). There is a significant increase in the number of cells engulfing beads in samples associated with high ROS levels (P. damicornis p-value < 0.001; N. vectensis p-value < 0.01). (B) Comparison of low and high levels of lysolitic vesicle signaling in P. damicornis (blue bars) and N. vectensis (green bars). There is a significant increase in the number of cells engulfing beads in samples associated with high lysosomal levels (P. damicornis p-value < 0.001; N. vectensis p-value < 0.001). P-value marks: **p < 0.01, ***p < 0.001.
Similar articles
-
Heat stress increases immune cell function in Hexacorallia.
Eliachar S, Snyder GA, Barkan SK, Talice S, Otolenghi A, Jaimes-Becerra A, Sharoni T, Sultan E, Hadad U, Levy O, Moran Y, Gershoni-Yahalom O, Traylor-Knowles N, Rosental B. Eliachar S, et al. Front Immunol. 2022 Dec 22;13:1016097. doi: 10.3389/fimmu.2022.1016097. eCollection 2022. Front Immunol. 2022. PMID: 36618389 Free PMC article.
-
Phagocytosis--the mighty weapon of the silent warriors.
Djaldetti M, Salman H, Bergman M, Djaldetti R, Bessler H. Djaldetti M, et al. Microsc Res Tech. 2002 Jun 15;57(6):421-31. doi: 10.1002/jemt.10096. Microsc Res Tech. 2002. PMID: 12112425 Review.
-
Measuring Phagosome pH by Ratiometric Fluorescence Microscopy.
Nunes P, Guido D, Demaurex N. Nunes P, et al. J Vis Exp. 2015 Dec 7;(106):e53402. doi: 10.3791/53402. J Vis Exp. 2015. PMID: 26710109 Free PMC article.
-
Jiang S, Jia Z, Xin L, Sun Y, Zhang R, Wang W, Wang L, Song L. Jiang S, et al. Fish Shellfish Immunol. 2016 Aug;55:490-8. doi: 10.1016/j.fsi.2016.06.024. Epub 2016 Jun 20. Fish Shellfish Immunol. 2016. PMID: 27338208
-
Integrins and small GTPases as modulators of phagocytosis.
Sayedyahossein S, Dagnino L. Sayedyahossein S, et al. Int Rev Cell Mol Biol. 2013;302:321-54. doi: 10.1016/B978-0-12-407699-0.00006-6. Int Rev Cell Mol Biol. 2013. PMID: 23351714 Review.
Cited by
-
Heat stress increases immune cell function in Hexacorallia.
Eliachar S, Snyder GA, Barkan SK, Talice S, Otolenghi A, Jaimes-Becerra A, Sharoni T, Sultan E, Hadad U, Levy O, Moran Y, Gershoni-Yahalom O, Traylor-Knowles N, Rosental B. Eliachar S, et al. Front Immunol. 2022 Dec 22;13:1016097. doi: 10.3389/fimmu.2022.1016097. eCollection 2022. Front Immunol. 2022. PMID: 36618389 Free PMC article.
-
Mandujano-Tinoco EA, Sultan E, Ottolenghi A, Gershoni-Yahalom O, Rosental B. Mandujano-Tinoco EA, et al. Cells. 2021 Jul 22;10(8):1853. doi: 10.3390/cells10081853. Cells. 2021. PMID: 34440622 Free PMC article. Review.
-
The Skeleton and Biomineralization Mechanism as Part of the Innate Immune System of Stony Corals.
Levy S, Mass T. Levy S, et al. Front Immunol. 2022 Feb 25;13:850338. doi: 10.3389/fimmu.2022.850338. eCollection 2022. Front Immunol. 2022. PMID: 35281045 Free PMC article. Review.
-
Structural and Evolutionary Relationships of Melanin Cascade Proteins in Cnidarian Innate Immunity.
Van Buren EW, Ponce IE, Beavers KM, Stokes A, Cornelio MN, Emery M, Mydlarz LD. Van Buren EW, et al. Integr Comp Biol. 2024 Nov 21;64(5):1320-1337. doi: 10.1093/icb/icae115. Integr Comp Biol. 2024. PMID: 39025801 Free PMC article.
-
Toullec G, Rädecker N, Pogoreutz C, Banc-Prandi G, Escrig S, Genoud C, Olmos CM, Spangenberg J, Meibom A. Toullec G, et al. Microbiome. 2024 Feb 29;12(1):42. doi: 10.1186/s40168-023-01738-0. Microbiome. 2024. PMID: 38424629 Free PMC article.
References
Publication types
MeSH terms
Substances
LinkOut - more resources
Full Text Sources