GIV/Girdin, a non-receptor modulator for Gαi/s, regulates spatiotemporal signaling during sperm capacitation and is required for male fertility - PubMed
- ️Fri Jan 01 2021
GIV/Girdin, a non-receptor modulator for Gαi/s, regulates spatiotemporal signaling during sperm capacitation and is required for male fertility
Sequoyah Reynoso et al. Elife. 2021.
Abstract
For a sperm to successfully fertilize an egg, it must first undergo capacitation in the female reproductive tract and later undergo acrosomal reaction (AR) upon encountering an egg surrounded by its vestment. How premature AR is avoided despite rapid surges in signaling cascades during capacitation remains unknown. Using a combination of conditional knockout (cKO) mice and cell-penetrating peptides, we show that GIV (CCDC88A), a guanine nucleotide-exchange modulator (GEM) for trimeric GTPases, is highly expressed in spermatocytes and is required for male fertility. GIV is rapidly phosphoregulated on key tyrosine and serine residues in human and murine spermatozoa. These phosphomodifications enable GIV-GEM to orchestrate two distinct compartmentalized signaling programs in the sperm tail and head; in the tail, GIV enhances PI3K→Akt signals, sperm motility and survival, whereas in the head it inhibits cAMP surge and premature AR. Furthermore, GIV transcripts are downregulated in the testis and semen of infertile men. These findings exemplify the spatiotemporally segregated signaling programs that support sperm capacitation and shed light on a hitherto unforeseen cause of infertility in men.
Keywords: Girdin; Sperm; cAMP; cell biology; developmental biology; human; male fertility; mouse; spermatozoa.
© 2021, Reynoso et al.
Conflict of interest statement
SR none, VC, GK, IL, ST, CE, CR, DS, PG, PG None
Figures
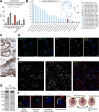
(A) Bar graph displays the relative fluorescence unit (RFU) of endogenous full-length GIV protein in immunoblots of organ lysates published previously using three independent anti-GIV antibodies raised against different epitopes of GIV (Anai et al., 2005). (Figure 1—source data 1)(B) RNA expression in the single-cell-type clusters identified in the human testis visualized by a UMAP plot (inset) and a bar plot. The bar plot shows RNA expression (pTPM) in each cell-type cluster. UMAP plot visualizes the cells in each cluster, where each dot corresponds to a cell. (C) Representative images from human testis immunistochemistry studies curated in the Human Protein Atlas. Int: interstitium; Lu: lumen of seminiferous tubules. (D) Cryosections of mouse testis (8 weeks old, C57BL/6) were stained for either total GIV (tGIV; green) and DAPI (blue, nucleus) alone, or co-stained with tGIV and the sperm acrosomal matrix protein zona pellucida 3 receptor (ZP3R, formerly called sp56; red) and analyzed by confocal immunofluorescence. Representative images from two independent analyses are displayed. Scale bar = 10 µm. (E, F) Cryosections of mouse testis tissue analyzed for total (t) GIV (green), pY GIV (red), and DAPI (blue, nucleus). Representative images from two independent analyses are shown in panel (E); scale bar = 10 µm. Insets in panel (E) are magnified and displayed in panel (F, left). Schematics in panel (F, right) display various localization of GIV observed during the process of maturation of the Golgi into acrosomal cap. (G) Immunoblots on mouse testis lysates with the same tGIV and pY GIV antibodies. (Figure 1—source data 2).
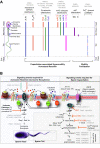
(A) The localization of G protein subunits and membrane and soluble adenylyl cyclases (mAC/sAC) and the calcium channel, Catsper, is shown. The intensity of the bar denotes the relative concentrations of the molecules. (B) Summary of experimental evidence and key citations and unknown (?) aspects in spatiotemporally separated signaling cascades that regulate sperm processes.
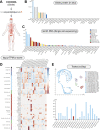
(A) CCDC88A expression profile was queried in the Human Protein Atlas. (B) GIV protein expression data is shown for each of the 44 tissues. (C) A summary of single-cell RNA (NX) from all single-cell types. Color coding is based on cell-type groups, each consisting of cell types with functional features in common. (D) The heatmap in this section shows expression of CCDC88A and well-known cell-type markers in the different single-cell-type clusters of human testes. Normalized data is presented as log (p-TPM) Z-score. (E) UMAP plot (top) for single-cell expression of CCDC88A in human testes. A bar chart (bottom) shows RNA expression (pTPM) in each cell-type cluster.
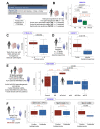
(A) Schematic displays the approach used to search NCBI GEO database for testis and sperm transcriptomic datasets suitable to study correlations between the abundance of CCDC88A transcripts and male fertility. (B–E) Whisker plots show the relative abundance of CCDC88A (expressed as Log2 normalized expression; see Materials and methods for different normalization approaches used for microarray and RNA-seq datasets) in sperm or testis samples (as annotated using schematics) in samples annotated with fertility status, or syndromes associated with infertility. (F) Whisker plots show the relative abundance of CCDC88A transcripts in sperms classified as subfertile or not based on three properties of sperm assessed using a modified WHO criterion published by Guzick et al., 2001 (see Materials and methods). Distribution of gene expression values is illustrated using boxplots and mean as circle with 95% confidence intervals (CIs) as arrows. Numbers on top indicate the p values, which were derived from Welch’s t-test. A significance level of <0.05, corresponding to 95% CIs are indicated in black font. Insignificant p values are indicated in red font.
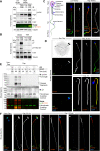
(A) Freshly ejaculated human sperm were segregated into low-motile and high-motile populations using ‘swim-up’ technique (see Materials and methods) and subsequently capacitated in vitro for 1 or 4 hr prior to whole cell lysis. Equal aliquots of lysates were analyzed by immunoblotting for total (t) GIV, pan pY, pY1764 GIV (pYGIV), and β-tubulin (loading control) using LI-COR Odyssey (Figure 3—source data 1). (B) Whole-cell lysates of human sperms capacitated with or without preincubation with H89 (protein kinase A [PKA] inhibitor) or DMSO control were analyzed as in (A) (Figure 3—source data 2). (C, D) Human sperm with low vs. high motility (C), were capacitated or not (D), fixed and co-stained for total and pY GIV (tGIV; pY GIV), tubulin and DAPI. Representative images that capture the most frequently observed staining patterns (at >80% frequency) among ~100–150 sperms/sample, in three independent samples, derived from three unique subjects are shown. Scale bar = 10 µm. (E) Immunoblots of equal aliquots of whole-cell lysates of mouse sperm capacitated with (+) or without (-) pretreatment with PKA inhibitor (H89) or vehicle (DMSO) control. Hexokinase is used as a loading control (Figure 3—source data 3). (F) Non-capacitated (non-cap) or capacitated mouse sperm were fixed and stained as in (D) and analyzed by confocal microscopy. Representative images that capture the most frequently observed staining patterns (at >80% frequency) among ~50–100 sperms/sample, in three independent samples, derived from three mice are shown. Scale bar = 10 µm.
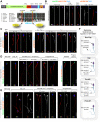
(A) Schematic shows the domain map of GIV (top) and the evolutionarily conserved GEM motif within its C terminus. A functional GEM motif is required for GIV to bind and activate Gαi as well as bind and inhibit Gαs (Gupta et al., 2016). Important phosphoserine modifications that regulate GIV’s GEM motif and the corresponding target kinases are highlighted. (B, C) Non-capacitated and capacitated mouse (B) and human (C) sperm were fixed and analyzed for the phosphoserine modifications highlighted in (A). (D, E) Mouse sperm with/without capacitation followed by treatment with either Ca2+ ionophore or progesterone to trigger AR were fixed and co-stained for peanut agglutinin (PNA-488; green, an acrosomal marker) and either pYGIV (D) or pSerGIV (E) and DAPI. Representative images are shown. Scale bar = 10 µm. (F) Schematic summarizes the spatially segregated phosphomodifications on GIV before and after capacitation and AR in various parts of the sperm. (i) Inhibitory phosphorylation at pS1689 on GIV is seen in both head and tail prior to capacitation (F, top); (ii) activating phosphorylation at S1674 on GIV is seen in the sperm head and tail, whereas pYGIV is predominantly seen in the mid-piece and the tail regions upon capacitation (post-cap; F) as well as during AR before the acrosome is shed (pre-AR; F); and (iii) after the acrosome is shed, pYGIV is the only phospho-GIV that is detected, and predominantly in the mid-piece (post-AR; F). Representative images that capture the most frequently observed staining pattern(s) (at >80% frequency), among ~50–150 sperms/sample, three independent samples, derived either from human subjects (n = 3) or mice (n = 3) are shown.
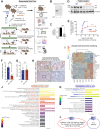
(A) Schematic showing the workflow for fertility studies in conditional GIV-cKO mice. After intraperitoneal injection of tamoxifen, male mice were first primed in two phases—first by co-housing with female littermates × 3 weeks, and subsequently by co-housing with female mice from Jackson laboratory (JAX) while the females acclimatized to the animal facility. The final ‘test’ group consisted of tamoxifen-injected WT and GIV-cKO male mice randomly assigned to and co-housed with three female mice from JAX, each with proven ability to get pregnant. (B–D) Confirmation of GIV-cKO in the mice after tamoxifen injection by genotyping (B), qPCR of testis tissues (C), and immunoblotting of testis lysates (D) (Figure 5—source data 1). (E) Kaplan–Meier plot showing the cumulative probability of pregnancy (expressed as %) in the females co-housed with either WT or GIV-cKO males. Statistical significance was assessed using log-rank analysis. *p<0.05 (Figure 5—source data 2). (F, G) Bar graphs showing the average litter size (F; Figure 5—source data 3) and sperm count (G; Figure 5—source data 4) in WT and GIV-cKO males. See also Figure 5—figure supplement 1 for quantifications of tested weight and length. (H) Immunohistochemistry staining on mouse testis. Scale bar = 200 µm. (I) Unsupervised clustering of WT and KO testis samples based on gene expression. Differentially expressed genes (DEGs) that were up- or downregulated in KO are annotated on the right side (Figure 5—source data 5). (J, K) Reactome pathway analyses showing the pathways that are up or downregulated in KO testis. (L) Summary of the most prominent conclusions from RNA-seq dataset.
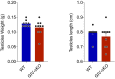
GIV was depleted in male mice by intraperitoneal injection of tamoxifen (see Figure 5A). Bar graphs show the testes weight (left; Figure 5—figure supplement 1—source data 1) and length (right; Figure 5—figure supplement 1—source data 2) in WT and GIV-cKO males. All results are presented as average ± SEM. Statistical significance was assessed using unpaired t-test, non-significant p-values>0.05.
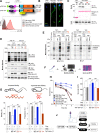
(A, B) Schematic (A, top) of cell-permeant His-TAT-GIV-CT wildtype (WT) and GEM-deficient mutant (F1685A; FA) peptides used in this work. Immunofluorescence images (B) representative of sperms after treatment with cell-permeant TAT-GIV-CT peptides and stained with anti-His antibody and DAPI. Scale bar = 15 µm. Histograms (A, bottom) from flow cytometry studies conducted with or without permeabilization confirm the uptake of His-TAT peptides in sperm. (C) Immunoblots of GST pulldown assays testing the ability of GDP-loaded GST-Gαi3 to bind TAT-GIVCT peptides from lysates of sperms at 1 hr (B1) and 6 hr (B2) after transduction (Figure 6—source data 1). (D, E) Immunoblots of lysates of TAT-GIVCT-transduced sperms at the indicated time points after capacitation analyzed for phospho-PKA substrates (D), phospho(p) and total (t) Akt (D; Figure 6—source data 2), pYGIV (E, left), pan-pY (E, right) (Figure 6—source data 3), and hexokinase (loading control, D). (F–I) Schematic in (F) summarizes workflow in assessing motility and survival of sperms during capacitation. Bar graphs in (G; Figure 6—source data 4) display the relative % of motile and progressively motile population of sperms. Line graphs in (H) show survival of sperms as determined by methy thiazolyl tetrazolium (MTT) assay; bar graphs in (I) show the area under the curve (AUC) of the line graphs in (H) (Figure 6—source data 5). All results are presented as average ± SEM of three independent studies conducted on sperm isolated from three mice. Statistical significance was assessed using one-way analysis of variance (ANOVA) followed by Tukey’s test for multiple comparisons. *p<0.05, ***p<0.001, ****p<0.0001, ns p>0.05. (J) Schematic summarizes the conclusions of how GIV’s GEM function impacts sperm phenotypes during capacitation.
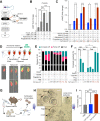
(A) Schematic summarizes the current knowledge of how Ca2+ and cAMP signaling regulates acrosome exocytosis during AR and how GIV’s ability to modulate cAMP via both Gαi/s is hypothesized to impact AR. (B) Bar graphs display the fold change in cAMP in mouse sperms treated with various stimuli in the presence of DMSO. All results are presented as average ± SEM of three independent studies conducted on sperm isolated from three mice. Statistical significance was assessed using one-way analysis of variance (ANOVA) followed by Tukey’s test for multiple comparisons. nsp>0.05, ****p<0.0001. (C) Bar graphs display the fold change in cAMP in TAT-GIVCT-transduced mouse sperms exposed to various stimuli. Dotted horizontal line represents cAMP concentration in PBS-treated samples, to which all other values were normalized. See also Figure 7—figure supplement 1 for comparison of PBS vs. all other treatments and conditions with (+) or without (-) peptides. All results are presented as average ± SEM of three independent studies conducted on sperm isolated from three mice. Statistical significance was assessed using two-way ANOVA followed by Sidak’s test for multiple comparisons. *p<0.05, ***p<0.001, ****p<0.0001, nsp>0.05 (Figure 7—source data 1). (D) Schematic on top summarizes the assay used to quantify progressive changes in acrosome membrane during AR that was induced in vitro by exposing capacitated sperms to 10 µM A23186 or 100 µM progesterone. Images in the bottom panel are representative of acrosome-intact, partial AR and complete AR stages. (E, F) Stacked bar graphs in (E) display the proportion of sperms in each indicated condition that are either in partial or complete AR or with intact acrosomes. Bar graphs in (F) display just the relative proportion of sperms in (E) that have complete AR. All results are presented as average ± SEM of three independent studies conducted on sperm isolated from three mice. Statistical significance was assessed using one-way ANOVA followed by Tukey’s test for multiple comparisons. *p<0.05, **p<0.01, ****p<0.0001 (Figure 7—source data 2). (G–I) Schematic in (G) displays the workflow used for in vitro fertilization (IVF) assays in (H, I). Representative images in (H) display the two-cell stage, which is quantified as % of total eggs in the assay and displayed as bar graphs in (I) as an indication of successful fertilization. Results are presented as average ± SEM of three independent studies conducted on sperm isolated from three mice. Statistical significance was assessed using one-way ANOVA including a Tukey’s test for multiple comparisons. ****p<0.0001, nsp>0.05 (Figure 7—source data 3).
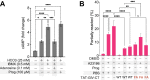
(A) Bar graphs display the fold change in cAMP in mouse sperms in the presence (+) or absence (-) of various treatments (Figure 7—figure supplement 1—source data 1). (B) Acrosomal reaction was analyzed in TAT-GIVCT-transduced mouse sperms exposed to either DMSO control or the calcium ionophore A23186 or progesterone using CD46 as a marker of inner acrosomal membrane (IAM) as outlined in Figure 7D. Bar graph presented here shows the proportion of partially reacted sperms in each treatment group. All results are presented as average ± SEM. Statistical significance was assessed using one-way analysis of variance (ANOVA) followed by Tukey’s test for multiple comparisons. *p<0.05, **p<0.01, ***p<0.001, ****p<0.0001 (Figure 7—source data 2).
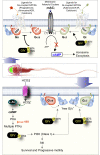
Schematic summarizes the key findings in this work and places them in the context of existing literature. GIV is likely to primarily function during capacitation of sperm, during which it fulfills two key roles as a signal transducer in a spatiotemporally segregated manner. The first role (right, top) is in the head of the sperm, where GIV’s GEM motif inhibits the AC→cAMP pathway and prevents acrosomal reaction. The second role (right, bottom) is in the mid-piece and tail region of the sperm, which involves tyrosine phosphorylation of GIV, which happens downstream of PKA activation. Such phosphorylation is rapidly induced during capacitation. In addition, GIV’s GEM motif is activated and is required for the enhancement of PI3K/Akt signals, enhanced motility, and survival of sperms during capacitation.
Similar articles
-
Protein kinase C-theta (PKCθ) phosphorylates and inhibits the guanine exchange factor, GIV/Girdin.
López-Sánchez I, Garcia-Marcos M, Mittal Y, Aznar N, Farquhar MG, Ghosh P. López-Sánchez I, et al. Proc Natl Acad Sci U S A. 2013 Apr 2;110(14):5510-5. doi: 10.1073/pnas.1303392110. Epub 2013 Mar 18. Proc Natl Acad Sci U S A. 2013. PMID: 23509302 Free PMC article.
-
Ear J, Abd El-Hafeez AA, Roy S, Ngo T, Rajapakse N, Choi J, Khandelwal S, Ghassemian M, McCaffrey L, Kufareva I, Sahoo D, Ghosh P. Ear J, et al. J Biol Chem. 2021 Jan-Jun;296:100493. doi: 10.1016/j.jbc.2021.100493. Epub 2021 Mar 3. J Biol Chem. 2021. PMID: 33675748 Free PMC article.
-
GIV/Girdin activates Gαi and inhibits Gαs via the same motif.
Gupta V, Bhandari D, Leyme A, Aznar N, Midde KK, Lo IC, Ear J, Niesman I, López-Sánchez I, Blanco-Canosa JB, von Zastrow M, Garcia-Marcos M, Farquhar MG, Ghosh P. Gupta V, et al. Proc Natl Acad Sci U S A. 2016 Sep 27;113(39):E5721-30. doi: 10.1073/pnas.1609502113. Epub 2016 Sep 12. Proc Natl Acad Sci U S A. 2016. PMID: 27621449 Free PMC article.
-
Harayama H. Harayama H. J Reprod Dev. 2013 Oct;59(5):421-30. doi: 10.1262/jrd.2013-056. J Reprod Dev. 2013. PMID: 24162806 Free PMC article. Review.
-
Peroxisome proliferator-activated receptor gamma signaling in human sperm physiology.
Liu LL, Xian H, Cao JC, Zhang C, Zhang YH, Chen MM, Qian Y, Jiang M. Liu LL, et al. Asian J Androl. 2015 Nov-Dec;17(6):942-7. doi: 10.4103/1008-682X.150253. Asian J Androl. 2015. PMID: 25851655 Free PMC article. Review.
Cited by
-
Heterotrimeric G protein signaling without GPCRs: The Gα-binding-and-activating (GBA) motif.
Garcia-Marcos M. Garcia-Marcos M. J Biol Chem. 2024 Mar;300(3):105756. doi: 10.1016/j.jbc.2024.105756. Epub 2024 Feb 15. J Biol Chem. 2024. PMID: 38364891 Free PMC article. Review.
-
Small-molecule targeting of GPCR-independent noncanonical G-protein signaling in cancer.
Zhao J, DiGiacomo V, Ferreras-Gutierrez M, Dastjerdi S, Ibáñez de Opakua A, Park JC, Luebbers A, Chen Q, Beeler A, Blanco FJ, Garcia-Marcos M. Zhao J, et al. Proc Natl Acad Sci U S A. 2023 May 2;120(18):e2213140120. doi: 10.1073/pnas.2213140120. Epub 2023 Apr 25. Proc Natl Acad Sci U S A. 2023. PMID: 37098067 Free PMC article.
References
-
- Anai M, Shojima N, Katagiri H, Ogihara T, Sakoda H, Onishi Y, Ono H, Fujishiro M, Fukushima Y, Horike N, Viana A, Kikuchi M, Noguchi N, Takahashi S, Takata K, Oka Y, Uchijima Y, Kurihara H, Asano T. A novel protein kinase B (PKB)/AKT-binding protein enhances PKB kinase activity and regulates DNA synthesis. Journal of Biological Chemistry. 2005;280:18525–18535. doi: 10.1074/jbc.M500586200. - DOI - PubMed
-
- Balestrini PA, Sanchez-Cardenas C, Luque GM, Baro Graf C, Sierra JM, Hernández-Cruz A, Visconti PE, Krapf D, Darszon A, Buffone MG. Membrane hyperpolarization abolishes calcium oscillations that prevent induced acrosomal exocytosis in human sperm. FASEB Journal. 2021;35:e21478. doi: 10.1096/fj.202002333RR. - DOI - PubMed
-
- Bhandari D, Lopez-Sanchez I, To A, Lo I-C, Aznar N, Leyme A, Gupta V, Niesman I, Maddox AL, Garcia-Marcos M, Farquhar MG, Ghosh P. Cyclin-Dependent kinase 5 activates guanine nucleotide exchange factor GIV/girdin to orchestrate migration–proliferation dichotomy. PNAS. 2015;112:E4874–E4883. doi: 10.1073/pnas.1514157112. - DOI - PMC - PubMed
Publication types
MeSH terms
Substances
Grants and funding
LinkOut - more resources
Full Text Sources
Molecular Biology Databases
Research Materials