Increasing large wildfires over the western United States linked to diminishing sea ice in the Arctic - PubMed
- ️Fri Jan 01 2021
Increasing large wildfires over the western United States linked to diminishing sea ice in the Arctic
Yufei Zou et al. Nat Commun. 2021.
Abstract
The compound nature of large wildfires in combination with complex physical and biophysical processes affecting variations in hydroclimate and fuel conditions makes it difficult to directly connect wildfire changes over fire-prone regions like the western United States (U.S.) with anthropogenic climate change. Here we show that increasing large wildfires during autumn over the western U.S. are fueled by more fire-favorable weather associated with declines in Arctic sea ice during preceding months on both interannual and interdecadal time scales. Our analysis (based on observations, climate model sensitivity experiments, and a multi-model ensemble of climate simulations) demonstrates and explains the Arctic-driven teleconnection through regional circulation changes with the poleward-shifted polar jet stream and enhanced fire-favorable surface weather conditions. The fire weather changes driven by declining Arctic sea ice during the past four decades are of similar magnitude to other leading modes of climate variability such as the El Niño-Southern Oscillation that also influence fire weather in the western U.S.
© 2021. Battelle Memorial Institute.
Conflict of interest statement
The authors declare no competing interests.
Figures
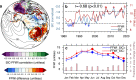
a Spatial distributions of the correlation (shading in the Arctic as denoted by the purple-green color bar) between seasonal average Arctic sea-ice concentrations (SIC) in summer and autumn (July to October) and a seasonal and regional average fire weather index (FFWI) over the western U.S. in the following autumn and early winter (September to December), and the difference of seasonal average FFWI (shading in North America as denoted by the blue-red color bar) between the years with minimum (SIC−: red up-pointing triangles in b) and maximum (SIC+: blue down-pointing triangles in b) Arctic SIC. The difference of seasonal (September to December) average geopotential height at 500 hPa between the SIC− and SIC+ years is also shown in a (contours with negative values in dashed lines; unit: m). Stipples in a mark regions that are significantly different from 0 at the 0.05 significance level of two-sided t-tests, and hatching in a denotes statistically significant regions based on the stricter FDR method (see Methods section) with local gridded p-value ≤pFDR*=0.0023 at the threshold of αFDR=0.10. b Time series of seasonal and regional average SIC (seasonal mean from July to October; normalized by its 1981–2010 climatological mean and standard deviation; note its scale on the left Y-axis is inverted to directly compare temporal variations of both time series), FFWI (seasonal mean from September to December), and their correlation. The region definitions for the Pacific sector of the Arctic and the western U.S. are outlined by the red and cyan boxes in a, respectively. The horizontal dashed lines denote the ±1 standard deviations of normalized SIC as thresholds for selecting the SIC± years. c The composite of monthly FFWI (solid lines with dots and error bars) and fractional burned area change of large wildfires (vertical bars) over the western U.S. Error bars in c denote ±1 standard deviations of monthly FFWI in each group. Dot sizes for monthly FFWI in c Denote the 0.05 (large),0.1 (medium), and non-significant (small) significance levels of two-sided t-tests for monthly FFWI differences between the years with minimum (FFWI_SIC−) and maximum (FFWI_SIC+) Arctic SIC, respectively.
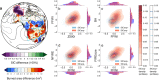
a Spatial distributions of the seasonal average (July to October) sea-ice concentration (SIC; unit: 100%) difference (color shading in the Arctic Ocean as denoted by the purple-green color bar) between the SICexp− and SICexp+ experiments, and the seasonal average (September to December) burned area difference (color shading in North America as denoted by the blue-red color bar) in response to the sea-ice perturbation. The difference of geopotential height at 500 hPa (Z500; unit: m) between SICexp− and SICexp+ is also shown (contours with negative values in dashed lines). The region definitions for the Pacific sector of the Arctic and the western U.S. are outlined by the red and cyan boxes, respectively. Stipples in a show regions that are significantly different from 0 at the 0.1 significance level of two-sided t-tests, and hatching in a denotes statistically significant regions based on the stricter FDR method (see Methods section) with local gridded p-value ≤pFDR*=0.017 at the threshold of αFDR=0.20. b Two-dimensional joint distributions of the seasonal mean fire-favorable circulation index (Z500i; standardized by first removing the 40-year mean and then normalizing by the standard deviation of Z500i from the SICexp+ experiment; unitless) and fire weather index (FFWI; also standardized by the 40-year mean and standard deviation of FFWI from the SICexp+ experiment; unitless) based on the kernel density estimation (KDE) for SICexp− (red shading) and SICexp+ (blue contours). The legends for color shading and contours are attached aside, and corresponding 1-d KDE distributions for each index in SICexp− (red) and SICexp+ (blue) are also shown along the x- and y-axis. c As in b, but for the comparison of FFWI and regional total burned area (BA; unitless) that are both standardized. d As in b, but for the comparison of FFWI and regional mean fire occurrence (NFIRE; unitless) that are both standardized. e As in b, but for the comparison of FFWI and regional mean fire size (FIREsize; unitless) that are both standardized. Note that the model-based fire variables are averaged over the coarse model grid cells that describe statistical properties of fire ensembles at each grid cell rather than individual properties of each single fire.
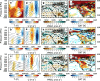
a Zonally averaged (170 °W to 60 °W; as shown in g) temperature (T; color shading; unit: K) difference in autumn and early winter (September to December) between the years with minimum (SIC−) and maximum sea-ice concentration (SIC+) based on the original ERA5 reanalysis data. The time average of zonally averaged temperature in the SIC+ years is also shown (contours; unit: K). b As in a, but for the temperature difference between the years with minimum (SICnotrd−) and maximum sea-ice concentration (SICnotrd+) based on the detrended ERA5 reanalysis data. c As in a, but for the temperature difference between the experiments with minimum (SICexp−) and maximum sea-ice concentration (SICexp+) based on the CESM-RESFire simulations. d–f As in a–c, but for zonally averaged zonal wind (U; color shading; unit: m s−1) difference based on the original ERA5 reanalysis data, the detrended ERA5 reanalysis data, and the CESM-RESFire simulations, respectively. g–i As in a–c, but for wind circulation at 500 hPa (arrows; unit: m s−1) and total precipitation rate (PREC; color shading; unit: mm d−1) differences based on the original ERA5 reanalysis data, the detrended ERA5 reanalysis data, and the CESM-RESFire simulations, respectively. j–l As in a–c, but for surface relative humidity (cyan contours with negative values in dashed lines; unit: %) and surface air temperature (SAT; color shading; unit: K) differences based on the original ERA5 reanalysis data, the detrended ERA5 reanalysis data, and the CESM-RESFire simulations, respectively. Stipples in a–l show regions that are significantly different from 0 at the 0.1 significance level of a two-sided t-test.
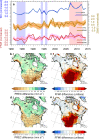
a Time series of normalized seasonal and regional average sea-ice concentration (SIC; unitless), a fire-favorable circulation index (Z500i; unitless), and a fire weather index (FFWI; unitless) based on the observational/reanalysis data and 15 amip model ensemble. The shading along lines of the time series denotes ±1 standard deviations of the model ensemble, and the vertical bar shading denotes years with minimum (pink; SIC−) and maximum (blue; SIC+) SIC for the composite differences in b and c. Note the SIC scale on the top left Y-axis is inverted for direct comparison with the other two variables. b The total difference in precipitation rates (PREC; color shading; unit: mm d−1) in autumn and early winter (September to December) between the SIC− and SIC+ years based on the 12 amip model ensemble. c As in b, but for the total difference in the fire weather index (FFWI; color shading; unitless). d The Arctic-driven (S/NP3) changes in precipitation rates (PREC; color shading; unit: mm d−1) in autumn and early winter (September to December) between the SIC− and SIC+ years based on the 12 amip model ensemble. e As in d, but for the Arctic-driven (S/NP3) changes in the fire weather index (FFWI; color shading; unitless) based on the 12 amip model ensemble. Stipples in b–e show regions that 2/3 amip models agree on the signs.
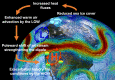
The L and H denote the cyclonic low pressure and anticyclonic high pressure circulation anomalies, respectively, induced by preceding Arctic sea-ice loss as suggested by the CESM-RESFire model sensitivity results shown in Fig. 2a. (Background image by NASA/Goddard Space Flight Center Scientific Visualization Studio).
Similar articles
-
ARCTIC CHANGE AND POSSIBLE INFLUENCE ON MID-LATITUDE CLIMATE AND WEATHER: A US CLIVAR White Paper.
Cohen J, Zhang X, Francis J, Jung T, Kwok R, Overland J, Ballinger T, Blackport R, Bhatt US, Chen H, Coumou D, Feldstein S, Handorf D, Hell M, Henderson G, Ionita M, Kretschmer M, Laliberte F, Lee S, Linderholm H, Maslowski W, Rigor I, Routson C, Screen J, Semmler T, Singh D, Smith D, Stroeve J, Taylor PC, Vihma T, Wang M, Wang S, Wu Y, Wendisch M, Yoon J. Cohen J, et al. US CLIVAR Rep. 2018 Mar;n/a:10.5065/D6TH8KGW. doi: 10.5065/D6TH8KGW. US CLIVAR Rep. 2018. PMID: 31633127 Free PMC article.
-
Shifting El Niño inhibits summer Arctic warming and Arctic sea-ice melting over the Canada Basin.
Hu C, Yang S, Wu Q, Li Z, Chen J, Deng K, Zhang T, Zhang C. Hu C, et al. Nat Commun. 2016 Jun 2;7:11721. doi: 10.1038/ncomms11721. Nat Commun. 2016. PMID: 27251873 Free PMC article.
-
Luo B, Luo D, Ge Y, Dai A, Wang L, Simmonds I, Xiao C, Wu L, Yao Y. Luo B, et al. Nat Commun. 2023 Feb 3;14(1):585. doi: 10.1038/s41467-023-36136-5. Nat Commun. 2023. PMID: 36737448 Free PMC article.
-
Climate and wildfires in the North American boreal forest.
Macias Fauria M, Johnson EA. Macias Fauria M, et al. Philos Trans R Soc Lond B Biol Sci. 2008 Jul 12;363(1501):2317-29. doi: 10.1098/rstb.2007.2202. Philos Trans R Soc Lond B Biol Sci. 2008. PMID: 18006414 Free PMC article. Review.
-
Climate of the Arctic marine environment.
Walsh JE. Walsh JE. Ecol Appl. 2008 Mar;18(2 Suppl):S3-22. doi: 10.1890/06-0503.1. Ecol Appl. 2008. PMID: 18494360 Review.
Cited by
-
Warm Arctic-Cold Eurasia pattern helps predict spring wildfire burned area in West Siberia.
Yin Z, Zhang Y, He S, Wang H. Yin Z, et al. Nat Commun. 2024 Oct 19;15(1):9041. doi: 10.1038/s41467-024-53470-4. Nat Commun. 2024. PMID: 39426984 Free PMC article.
-
Enhanced simulated early 21st century Arctic sea ice loss due to CMIP6 biomass burning emissions.
DeRepentigny P, Jahn A, Holland MM, Kay JE, Fasullo J, Lamarque JF, Tilmes S, Hannay C, Mills MJ, Bailey DA, Barrett AP. DeRepentigny P, et al. Sci Adv. 2022 Jul 29;8(30):eabo2405. doi: 10.1126/sciadv.abo2405. Epub 2022 Jul 27. Sci Adv. 2022. PMID: 35895816 Free PMC article.
-
Quantifying Exposure to Wildfire Smoke Among Schoolchildren in California, 2006 to 2021.
Velásquez EE, Benmarhnia T, Casey JA, Aguilera R, Kiang MV. Velásquez EE, et al. JAMA Netw Open. 2023 Apr 3;6(4):e235863. doi: 10.1001/jamanetworkopen.2023.5863. JAMA Netw Open. 2023. PMID: 37017969 Free PMC article.
-
Rapid summer Russian Arctic sea-ice loss enhances the risk of recent Eastern Siberian wildfires.
Luo B, Luo D, Dai A, Xiao C, Simmonds I, Hanna E, Overland J, Shi J, Chen X, Yao Y, Duan W, Liu Y, Zhang Q, Xu X, Diao Y, Jiang Z, Gong T. Luo B, et al. Nat Commun. 2024 Jun 26;15(1):5399. doi: 10.1038/s41467-024-49677-0. Nat Commun. 2024. PMID: 38926364 Free PMC article.
-
Ren L, Yang Y, Wang H, Wang P, Yue X, Liao H. Ren L, et al. Geophys Res Lett. 2022 Aug 16;49(15):e2022GL099308. doi: 10.1029/2022GL099308. Epub 2022 Aug 5. Geophys Res Lett. 2022. PMID: 35941985 Free PMC article.
References
-
- Dennison PE, Brewer SC, Arnold JD, Moritz MA. Large wildfire trends in the western United States, 1984-2011. Geophys. Res. Lett. 2014;41:2928–2933. doi: 10.1002/2014GL059576. - DOI
-
- Thomas, D., Butry, D., Gilbert, S., Webb, D., & Fung, J. The Costs and Losses of Wildfires: A Literature Survey (National Institute of Standards and Technology (NIST, 2017).
LinkOut - more resources
Full Text Sources
Miscellaneous