Modulating gene expression in breast cancer via DNA secondary structure and the CRISPR toolbox - PubMed
- ️Fri Jan 01 2021
Modulating gene expression in breast cancer via DNA secondary structure and the CRISPR toolbox
Jessica A Kretzmann et al. NAR Cancer. 2021.
Abstract
Breast cancer is the most commonly diagnosed malignancy in women, and while the survival prognosis of patients with early-stage, non-metastatic disease is ∼75%, recurrence poses a significant risk and advanced and/or metastatic breast cancer is incurable. A distinctive feature of advanced breast cancer is an unstable genome and altered gene expression patterns that result in disease heterogeneity. Transcription factors represent a unique therapeutic opportunity in breast cancer, since they are known regulators of gene expression, including gene expression involved in differentiation and cell death, which are themselves often mutated or dysregulated in cancer. While transcription factors have traditionally been viewed as 'undruggable', progress has been made in the development of small-molecule therapeutics to target relevant protein-protein, protein-DNA and enzymatic active sites, with varying levels of success. However, non-traditional approaches such as epigenetic editing, transcriptional control via CRISPR/dCas9 systems, and gene regulation through non-canonical nucleic acid secondary structures represent new directions yet to be fully explored. Here, we discuss these new approaches and current limitations in light of new therapeutic opportunities for breast cancers.
© The Author(s) 2021. Published by Oxford University Press on behalf of NAR Cancer.
Figures
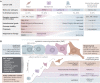
(A) Characteristics of breast cancer subtypes, based on collated data (241–245); ER, estrogen receptor, PR, progesterone receptor, PARPi, poly(ADP-ribose) polymerase (PARP) inhibitor. (B) Epithelial–mesenchymal transition showing key properties of epithelial and mesenchymal phenotypes, triggers and traits conferred by EMT in cancer. Adapted with permission from Springer Nature: Tam, W.L. and Weinberg, R.A. The epigenetics of epithelial–mesenchymal plasticity in cancer (2013). Nat. Med., 19, 1438–1449 (246).
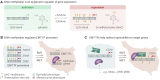
Key roles of DNAme in regulating gene expression in EMT. (A) DNA methylation of gene promoter CpG islands acts to repress gene expression. Methylation marks are deposited by DNA methyltransferase (DNMT), and removed by ten-eleven translocation (TET), families of enzymes. (B) Genes encoding EMT-TFs including TWIST, SNAIL (SNAI1) and ZEB2 are silenced by promoter methylation in epithelial cells. When these EMT-TFs are activated by EMT, they repress expression of epithelial genes, causing loss of cell adhesion and other epithelial phenotype characteristics. (C) EMT-TF expression can recruit epigenetic modifiers such as DNMT enzymes to methylate promoters of epithelial genes, resulting in stable and long-term repression. Adapted with permission from Elsevier: Skrypek, N., Goossens, S., De Smedt, E., Vandamme, N. and Berx, G. (2017) Epithelial-to-Mesenchymal Transition: Epigenetic Reprogramming Driving Cellular Plasticity. Trends Genet. TIG, 33, 943–959 (31).
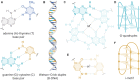
Schematic representation of DNA secondary structures. (A) Adenine–thymine and guanine–cytosine canonical Watson-Crick base pairing and (B) schematic representation of B-form DNA. (C) Planar G-tetrad structure formed through the self-association between four guanine bases via Hoogsteen hydrogen bonding, with a central monovalent cation shown within the center of the tetrad structure. (D) Schematic representation of an intramolecular G-quadruplex structure containing three stacked G-tetrads. (E) Hemi-protonated cytosine–cytosine base pairing, highlighting the N3 protonation of the cytosine ring. (F) Schematic representation of an intramolecular i-motif structure, through the self-association of several intercalated C···C+ base pairings.
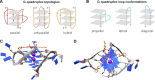
G-quadruplex topologies, loop conformations and example ligand binding modes. (A) G-quadruplex topologies for an intramolecular. (B) Loop conformations, highlighted in blue. (C) PhenDC3 ligand, shown in orange, interacts with a quadruplex via end-stacking and the grooves of this parallel G-quadruplex, PDB code: 3CE5. (D) Top-down view of the same structure as in (C).
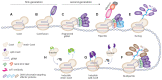
Schematic of the CRISPR/Cas9 toolbox. First-generation Cas9 systems include the (A) unmodified Cas9 system, and (B) the Cas9 enzyme fused to single effector proteins. Second-generation Cas9 systems (C–H) have evolved to enable either higher levels of modification, or more sophisticated mechanisms of controlling modification. The principle behind constructs aimed for higher levels of modification is to recruit multiple copies of effector proteins, which can be achieved through (C) engineering gRNA scaffold to include RNA aptamers (engineered sgRNA), (E) binding effectors along a repeating peptide array (Suntag), or (D and F) combinations thereof (tripartite and multipartite). Chemically inducible systems enable temporal control over the activity of the Cas9 fused effector proteins. (G) In the split-Cas9, each half of the Cas9 protein has to be induced to form the full, functional complex, while the intein-Cas9 approach (H) requires a protein segment to be excised after chemical induction. Adapted with permission from Adli et al. (2018) (207) under Creative Commons Attribution 4.0 International License (
https://creativecommons.org/licenses/by/4.0/).
Similar articles
-
Overview of resistance to systemic therapy in patients with breast cancer.
Gonzalez-Angulo AM, Morales-Vasquez F, Hortobagyi GN. Gonzalez-Angulo AM, et al. Adv Exp Med Biol. 2007;608:1-22. doi: 10.1007/978-0-387-74039-3_1. Adv Exp Med Biol. 2007. PMID: 17993229 Review.
-
A review on CRISPR/Cas-based epigenetic regulation in plants.
Jogam P, Sandhya D, Alok A, Peddaboina V, Allini VR, Zhang B. Jogam P, et al. Int J Biol Macromol. 2022 Oct 31;219:1261-1271. doi: 10.1016/j.ijbiomac.2022.08.182. Epub 2022 Aug 31. Int J Biol Macromol. 2022. PMID: 36057300 Review.
-
Medical Advisory Secretariat. Medical Advisory Secretariat. Ont Health Technol Assess Ser. 2010;10(23):1-57. Epub 2010 Dec 1. Ont Health Technol Assess Ser. 2010. PMID: 23074401 Free PMC article.
-
CRISPR/Cas9 in epigenetics studies of health and disease.
Sar P, Dalai S. Sar P, et al. Prog Mol Biol Transl Sci. 2021;181:309-343. doi: 10.1016/bs.pmbts.2021.01.022. Epub 2021 Mar 12. Prog Mol Biol Transl Sci. 2021. PMID: 34127198
-
CRISPR/Cas mediated epigenome editing for cancer therapy.
Ansari I, Chaturvedi A, Chitkara D, Singh S. Ansari I, et al. Semin Cancer Biol. 2022 Aug;83:570-583. doi: 10.1016/j.semcancer.2020.12.018. Epub 2021 Jan 6. Semin Cancer Biol. 2022. PMID: 33421620 Review.
Cited by
-
Editorial: NAR Cancer and epigenetics and cancer.
Yang D, Dickerhoff J, Dynan WS. Yang D, et al. NAR Cancer. 2022 Mar 4;4(1):zcac003. doi: 10.1093/narcan/zcac003. eCollection 2022 Mar. NAR Cancer. 2022. PMID: 35252865 Free PMC article. No abstract available.
-
G4-DNA formation and chromatin remodelling are interdependent in human cells.
Lawler NB, Ou A, King JJ, Evans CW, Iyer KS, Smith NM. Lawler NB, et al. Chem Sci. 2023 Jun 27;14(28):7681-7687. doi: 10.1039/d3sc02533k. eCollection 2023 Jul 19. Chem Sci. 2023. PMID: 37476710 Free PMC article.
-
Hydration water drives the self-assembly of guanosine monophosphate.
Tao YH, Schulke S, Schwaab G, Nealon GL, Pezzotti S, Hodgetts SI, Harvey AR, Havenith M, Wallace VP. Tao YH, et al. Biophys J. 2024 Apr 16;123(8):931-939. doi: 10.1016/j.bpj.2024.03.005. Epub 2024 Mar 7. Biophys J. 2024. PMID: 38454599
-
Emerging roles of i-motif in gene expression and disease treatment.
Luo X, Zhang J, Gao Y, Pan W, Yang Y, Li X, Chen L, Wang C, Wang Y. Luo X, et al. Front Pharmacol. 2023 Mar 20;14:1136251. doi: 10.3389/fphar.2023.1136251. eCollection 2023. Front Pharmacol. 2023. PMID: 37021044 Free PMC article. Review.
-
Ten Years of CRISPRing Cancers In Vitro.
Capoferri D, Filiberti S, Faletti J, Tavani C, Ronca R. Capoferri D, et al. Cancers (Basel). 2022 Nov 23;14(23):5746. doi: 10.3390/cancers14235746. Cancers (Basel). 2022. PMID: 36497228 Free PMC article. Review.
References
-
- Sung H., Ferlay J., Siegel R.L., Laversanne M., Soerjomataram I., Jemal A., Bray F. Global cancer statistics 2020: GLOBOCAN estimates of incidence and mortality worldwide for 36 cancers in 185 countries. CA Cancer J. Clin. 71:209–249. - PubMed
-
- Roser M., Ritchie H. Cancer. Our World in Data. 2015; https://ourworldindata.org/cancer.
LinkOut - more resources
Full Text Sources