Drying in the low-latitude Atlantic Ocean contributed to terrestrial water storage depletion across Eurasia - PubMed
- ️Sat Jan 01 2022
Drying in the low-latitude Atlantic Ocean contributed to terrestrial water storage depletion across Eurasia
Zexi Shen et al. Nat Commun. 2022.
Abstract
Eurasia, home to ~70% of global population, is characterized by (semi-)arid climate. Water scarcity in the mid-latitude Eurasia (MLE) has been exacerbated by a consistent decline in terrestrial water storage (TWS), attributed primarily to human activities. However, the atmospheric mechanisms behind such TWS decline remain unclear. Here, we investigate teleconnections between drying in low-latitude North Atlantic Ocean (LNATO) and TWS depletions across MLE. We elucidate mechanistic linkages and detecte high correlations between decreased TWS in MLE and the decreased precipitation-minus-evapotranspiration (PME) in LNATO. TWS in MLE declines by ~257% during 2003-2017 due to northeastward propagation of PME deficit following two distinct seasonal landfalling routes during January-May and June-January. The same mechanism reduces TWS during 2031-2050 by ~107% and ~447% under scenarios SSP245 and SSP585, respectively. Our findings highlight the risk of increased future water scarcity across MLE caused by large-scale climatic drivers, compounding the impacts of human activities.
© 2022. The Author(s).
Conflict of interest statement
The authors declare no competing interests.
Figures
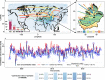
Contribution rates (CRs) of 500,000 water particles to the precipitation in Xinjiang during 2003–2017 by month, and cluster analysis of back traced trajectories of water particles (a). The routes of moisture trajectories and spatial pattern of terrain in Xinjiang (b). Temporal covariation of the total contribution rate (TCR) and the sum of CRs from Asia (AS), Europe (EU) and the North Atlantic Ocean (NATO) (CR(AS + EU + NATO)) during 2003–2017 (c). The time when CR(AS + EU + NATO)/TCR > 80% took account of 86.7% of the whole study period. The NXJ and SXJ refer to the north and south Xinjiang in the plot. The NAF in the subplot a refers to North Africa.
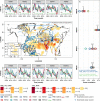
Temporal covariations among the normalized trend items of the regional sums of terrestrial water storages (TWSs) in sub-regions 1–10 (HSRs 1–10) across mid-latitude (N30°–N60°) Eurasia and the normalized trend items of the regional sums precipitation-minus-evapotranspiration (PMEs) in NATO1 and NATO3 (a–e and h–l, respectively). Spatial patterns of the modified Mann-Kendall (MMK) trends of PMEs in the NATO and TWSs in North Africa, Europe and Asia (g). Cross Pearson’s correlation between PMEs in northern NATOs and TWSs in HSRs 1–10 (f). PME_NATO_MMK refer to the MMK trend in PMEs over NATO. TWS_MMK refers to the MMK trend in TWS across Eurasia and North Africa. The ocean currents in the plot were the long-term averaged ocean currents in NATO based on the OSCAR (Ocean Surface Current Analysis Real-time) dataset during 1993–2019. The moisture trajectories in subplot (g) were clustered using the k-means cluster method based on the locations of particles in the trajectories.
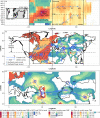
Longitudinal profile of monthly averaged mean precipitation-minus-evapotranspiration (PME) anomalies in the mid-latitude (N30°–N60°) Atlantic Ocean (NATO) and the monthly mean terrestrial water storage (TWSs) anomalies in mid-latitude (N30°–N60°) North Africa (NAF) and Eurasia from January to December (a). Spatial patterns of the sum of monthly mean PME anomalies (unit: mm) in NATO and the sum of negative monthly mean TWS anomalies (unit: mm) in lands during 2003–2017 (b). Transfer routes of the landfalling impacts of deficits in PMEs over northern NATO from ocean to land during 2003–2017 (c). The NXJ, SXJ, TM, HC, LP and NCP in subplot c refer to North Xinjiang, South Xinjiang, Tian Mountain, Hexi Corridor, Loess Plateau and North Chain Plain, respectively.
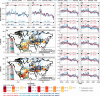
Temporal variations in the annual-sum normalized trend items of the zonal sums of TWSs across the whole HSR1-10 and variations in the annual-sum normalized trend items of zonal sums of PMEs in NATO1 and NATO3 under SSP245 and SSP585 during 2018–2050 (a, b). Spatial patterns of the modified Mann-Kendall (MMKs) trends of PME over NATO and TWS in HSR1-10 under SSP245 (c) and SSP585 (d) during 2018–2050 (c, d). Temporal variations in the annual-sum normalized trend item of the zonal sum of TWS over every HSR and annual-sum normalized trend items of the zonal sums of PMEs in NATO1 and NATO3 under SSP245 and SSP585 during 2018–2050, respectively (e–n). The VR in the plot refers to the varying rates of indices during the time interval, i.e., 2018–2031 and 2031–2050. The moisture trajectories in subplot (c, d) were categorized using the k-means cluster method based on the locations of water particles.
Similar articles
-
Oceanic climate changes threaten the sustainability of Asia's water tower.
Zhang Q, Shen Z, Pokhrel Y, Farinotti D, Singh VP, Xu CY, Wu W, Wang G. Zhang Q, et al. Nature. 2023 Mar;615(7950):87-93. doi: 10.1038/s41586-022-05643-8. Epub 2023 Mar 1. Nature. 2023. PMID: 36859582 Free PMC article.
-
Anyah RO, Forootan E, Awange JL, Khaki M. Anyah RO, et al. Sci Total Environ. 2018 Sep 1;635:1405-1416. doi: 10.1016/j.scitotenv.2018.04.159. Epub 2018 Apr 25. Sci Total Environ. 2018. PMID: 29710593
-
Tao F, Chen Y, Fu B. Tao F, et al. Sci Total Environ. 2020 Jul 1;724:138298. doi: 10.1016/j.scitotenv.2020.138298. Epub 2020 Mar 31. Sci Total Environ. 2020. PMID: 32272410
-
Obrist D, Kirk JL, Zhang L, Sunderland EM, Jiskra M, Selin NE. Obrist D, et al. Ambio. 2018 Mar;47(2):116-140. doi: 10.1007/s13280-017-1004-9. Ambio. 2018. PMID: 29388126 Free PMC article. Review.
-
Using Satellite-Based Terrestrial Water Storage Data: A Review.
Humphrey V, Rodell M, Eicker A. Humphrey V, et al. Surv Geophys. 2023;44(5):1489-1517. doi: 10.1007/s10712-022-09754-9. Epub 2023 Jan 13. Surv Geophys. 2023. PMID: 37771629 Free PMC article. Review.
Cited by
-
Enhanced dominance of soil moisture stress on vegetation growth in Eurasian drylands.
Zhang Y, Zhang Y, Lian X, Zheng Z, Zhao G, Zhang T, Xu M, Huang K, Chen N, Li J, Piao S. Zhang Y, et al. Natl Sci Rev. 2023 Apr 24;10(8):nwad108. doi: 10.1093/nsr/nwad108. eCollection 2023 Aug. Natl Sci Rev. 2023. PMID: 37389136 Free PMC article.
-
Oceanic climate changes threaten the sustainability of Asia's water tower.
Zhang Q, Shen Z, Pokhrel Y, Farinotti D, Singh VP, Xu CY, Wu W, Wang G. Zhang Q, et al. Nature. 2023 Mar;615(7950):87-93. doi: 10.1038/s41586-022-05643-8. Epub 2023 Mar 1. Nature. 2023. PMID: 36859582 Free PMC article.
References
-
- Pokhrel Y, et al. Global terrestrial water storage and drought severity under climate change. Nat. Clim. Chang. 2021;11:226–233.
-
- Gu X, et al. Intensification and expansion of soil moisture drying in warm season over Eurasia under global warming. J. Geophys. Res. Atmos. 2019;124:3765–3782.
-
- Zektser, I. S. & Everett, L. G. Groundwater Resources of the World and Their Use (UNESCO, Paris, 2004).
-
- Mueller ND. Closing yield gaps through nutrient and water management. Nature. 2012;490:254–257. - PubMed
Publication types
MeSH terms
Substances
LinkOut - more resources
Full Text Sources
Research Materials