Multi-omics data reveals the disturbance of glycerophospholipid metabolism caused by disordered gut microbiota in depressed mice - PubMed
Multi-omics data reveals the disturbance of glycerophospholipid metabolism caused by disordered gut microbiota in depressed mice
Tian Tian et al. J Adv Res. 2022 Jul.
Abstract
Introduction: Although researchers have done intensive research on depression, its pathogenesis is still not fully explained. More and more evidence suggests that gut microbiota is closely related to the onset of depression; but its specific functional ways are not clearly identified.
Objectives: The purpose of our work was to find out how the gut microbiota was involved in the onset of depression, and to identify the potential ways to link the gut and brain in mice with depressive-like behaviors (DLB).
Methods: We used the chronic restraint stress (CRS)-induced depression model here. Gut microbiota compositions in fecal samples, lipid metabolism (in fecal, serum and hippocampus samples) and neurotransmitters in hippocampus samples were detected.
Results: We found that the 7 of 13 differential genera that significantly correlated with DLB belonged to phylum Firmicutes. The differential lipid metabolites in fecal samples mainly belonged to glycerophospholipids (GP) and fatty acids (FA) metabolism, and three important "metabolite type-bacterial taxa" correlated pairs were identified: "FA/GP-Firmicutes", "FA/GP-Akkermansia", and "FA/GP-Bifidobacterium". The key differential lipid metabolites significantly correlated with DLB mainly belonged to FA and GP, and the DLB-related metagenomic genes were consistently enriched in GP metabolism and FA metabolism. Three significantly changed short-chain fatty acids (SCFAs) were significantly correlated with the majority of differential genera. Meanwhile, we found that the differential lipid metabolites in serum and hippocampus samples were mainly mapped into the GP metabolism, and there were four differential neurotransmitters from the tryptophan pathway in hippocampus samples.
Conclusion: Together, our findings could provide novel insights into the role of "microbiota-gut-brain" (MGB) axis in depression, and indicate that the gut microbiota might have a vital role in the onset of DLB by affecting the peripheral/central GP metabolism and tryptophan pathway. The "Firmicutes-SCFAs-GP metabolism-Tryptophan pathway" might be a possible way to link the gut and brain in depressed mice.
Keywords: Depression; Glycerophospholipids; Gut microbiota; Neurotransmitter.
Copyright © 2022. Production and hosting by Elsevier B.V.
Conflict of interest statement
Declaration of Competing Interest The authors declare that they have no known competing financial interests or personal relationships that could have appeared to influence the work reported in this paper.
Figures
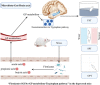
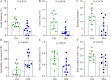
DLB in chronic restraint stress-induced depressed mice. A-C) DM showed the significantly decreased CD (%) (A), CT (%) (B) and RN (C) in OFT; D) the IT in FST was significantly higher in DM than in CM; E) the SPF in SPT was significantly decreased in DM than in CM; F) the body weight at 4 week was significantly lower in DM than in CM. DM, depressed mice; CM, control mice; CD, center distance; CT, center time; RN, the number of rearing; IT, immobility time; SPF, sucrose preference; OFT, open field test; FST, forced swimming test; SPT sucrose preference test.
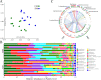
Disordered gut microbiota in depressed mice. A) The principal coordinate analysis showed the obvious differences in the gut microbial compositions between CM and DM; B) the relative abundance on Family level between CM and DM; C) the dominant bacteria taxa on Family level in the two groups. DM, depressed mice; CM, control mice.
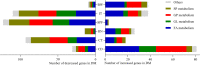
Correlations between differential genera and DLB. DM, depressed mice; CM, control mice; CD, center distance; IT, immobility time; SPF, sucrose preference; CT, center time; BW, body weight; RN, rearing number.
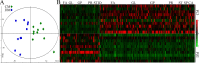
Disturbance of fecal lipid metabolism in DM. A) The orthogonal partial least-squares discriminant analysis showed the divergent metabolic phenotypes between CM and DM; B) heatmap of the identified differential lipid metabolites. DM, depressed mice; CM, control mice; GP, Glycerophospholipids; ID, Indoles and derivatives; GL, Glycerolipids; ST, Steroids and steroid derivatives; FA, Fatty acids; CA, Carboxylic acids and derivatives; SP, Sphingolipids; PR, Prenol lipids.
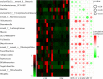
Differential lipid-related metagenomic genes correlated with DLB. CD, center distance; IT, immobility time; SPF, sucrose preference; CT, center time; BW, body weight; RN, rearing number; GP, Glycerophospholipids; FA, Fatty acids; GL, Glycerolipids; SP, Sphingolipids.
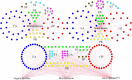
“Metabolite type-bacterial taxa” correlation pairs. GP, Glycerophospholipids; ID, Indoles and derivatives; GL, Glycerolipids; ST, Steroids and steroid derivatives; CA, Carboxylic acids and derivatives; FA, Fatty acids; SP, Sphingolipids; PR, Prenol lipids.
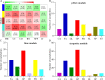
Metabolomic correlations with DLB. A) Heatmap of the correlation coefficients between DLB and metabolomic modules. Green and red squares indicated positive and negative correlation, respectively; B-D) the number of metabolites in each module that was significantly correlated with at least one kind of DLB. CD, center distance; IT, immobility time; SPF, sucrose preference; CT, center time; BW, body weight; RN, rearing number. GP, Glycerophospholipids; ID, Indoles and derivatives; GL, Glycerolipids; ST, Steroids and steroid derivatives; FA, Fatty acids; CA, Carboxylic acids and derivatives; SP, Sphingolipids; PR, Prenol lipids; SL, Saccharolipids.
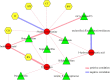
Correlations between differetial neurotransmitters, genera and behaviors. CD, center distance; IT, immobility time; SPF, sucrose preference; CT, center time; BW, body weight; RN, rearing number.
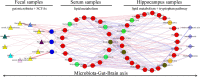
Possible ways connecting gut and brain in depressed mice.
Similar articles
-
Xie J, Zhong Q, Wu WT, Chen JJ. Xie J, et al. J Transl Med. 2023 Feb 7;21(1):93. doi: 10.1186/s12967-023-03942-w. J Transl Med. 2023. PMID: 36750892 Free PMC article.
-
Jiang W, Chen J, Gong L, Liu F, Zhao H, Yan Z, Li Y, Zhang J, Xiao M, Mu J. Jiang W, et al. J Psychiatr Res. 2023 May;161:426-434. doi: 10.1016/j.jpsychires.2023.03.032. Epub 2023 Apr 4. J Psychiatr Res. 2023. PMID: 37031497
-
Xie J, Wu WT, Chen JJ, Zhong Q, Wu D, Niu L, Wang S, Zeng Y, Wang Y. Xie J, et al. Front Cell Infect Microbiol. 2023 Feb 24;13:1121445. doi: 10.3389/fcimb.2023.1121445. eCollection 2023. Front Cell Infect Microbiol. 2023. PMID: 36909723 Free PMC article.
-
Interactions between polysaccharides and gut microbiota: A metabolomic and microbial review.
Zhang D, Liu J, Cheng H, Wang H, Tan Y, Feng W, Peng C. Zhang D, et al. Food Res Int. 2022 Oct;160:111653. doi: 10.1016/j.foodres.2022.111653. Epub 2022 Jul 10. Food Res Int. 2022. PMID: 36076442 Review.
-
The role of short-chain fatty acids in kidney injury induced by gut-derived inflammatory response.
Huang W, Zhou L, Guo H, Xu Y, Xu Y. Huang W, et al. Metabolism. 2017 Mar;68:20-30. doi: 10.1016/j.metabol.2016.11.006. Epub 2016 Nov 17. Metabolism. 2017. PMID: 28183450 Review.
Cited by
-
Hao W, Ma Q, Wang L, Yuan N, Gan H, He L, Li X, Huang J, Chen J. Hao W, et al. Microbiome. 2024 Feb 20;12(1):34. doi: 10.1186/s40168-024-01756-6. Microbiome. 2024. PMID: 38378622 Free PMC article.
-
Cheng J, Hu H, Ju Y, Liu J, Wang M, Liu B, Zhang Y. Cheng J, et al. Gen Psychiatr. 2024 Feb 19;37(1):e101374. doi: 10.1136/gpsych-2023-101374. eCollection 2024. Gen Psychiatr. 2024. PMID: 38390241 Free PMC article. Review.
-
Bu Q, Zhang J, Guo X, Feng Y, Yan H, Cheng W, Feng Z, Cao M. Bu Q, et al. Front Nutr. 2022 Sep 15;9:947697. doi: 10.3389/fnut.2022.947697. eCollection 2022. Front Nutr. 2022. PMID: 36185696 Free PMC article.
-
Zheng Y, Zhang L, He S, Xie Z, Zhang J, Ge C, Sun G, Huang J, Li H. Zheng Y, et al. BMJ Open. 2022 Nov 23;12(11):e067447. doi: 10.1136/bmjopen-2022-067447. BMJ Open. 2022. PMID: 36418119 Free PMC article.
-
Qian X, Hai W, Chen S, Zhang M, Jiang X, Tang H. Qian X, et al. Gut Microbes. 2023 Dec;15(2):2282790. doi: 10.1080/19490976.2023.2282790. Epub 2023 Nov 22. Gut Microbes. 2023. PMID: 37992400 Free PMC article.
References
-
- Mitchell A.J., Chan M., Bhatti H., Halton M., Grassi L., Johansen C., et al. Prevalence of depression, anxiety, and adjustment disorder in oncological, haematological, and palliative-care settings: a meta-analysis of 94 interview-based studies. Lancet Oncol. 2011;12(2):160–174. - PubMed
-
- Warden D., Rush A.J., Trivedi M.H., Fava M., Wisniewski S.R. The STAR*D Project results: a comprehensive review of findings. Curr Psychiatry Rep. 2007;9(6):449–459. - PubMed
Publication types
MeSH terms
Substances
LinkOut - more resources
Full Text Sources