Phenotypic and transcriptional response of Daphnia pulicaria to the combined effects of temperature and predation - PubMed
- ️Sat Jan 01 2022
Phenotypic and transcriptional response of Daphnia pulicaria to the combined effects of temperature and predation
Aaron Oliver et al. PLoS One. 2022.
Abstract
Daphnia, an ecologically important zooplankton species in lakes, shows both genetic adaptation and phenotypic plasticity in response to temperature and fish predation, but little is known about the molecular basis of these responses and their potential interactions. We performed a factorial experiment exposing laboratory-propagated Daphnia pulicaria clones from two lakes in the Sierra Nevada mountains of California to normal or high temperature (15°C or 25°C) in the presence or absence of fish kairomones, then measured changes in life history and gene expression. Exposure to kairomones increased upper thermal tolerance limits for physiological activity in both clones. Cloned individuals matured at a younger age in response to higher temperature and kairomones, while size at maturity, fecundity and population intrinsic growth were only affected by temperature. At the molecular level, both clones expressed more genes differently in response to temperature than predation, but specific genes involved in metabolic, cellular, and genetic processes responded differently between the two clones. Although gene expression differed more between clones from different lakes than experimental treatments, similar phenotypic responses to predation risk and warming arose from these clone-specific patterns. Our results suggest that phenotypic plasticity responses to temperature and kairomones interact synergistically, with exposure to fish predators increasing the tolerance of Daphnia pulicaria to stressful temperatures, and that similar phenotypic responses to temperature and predator cues can be produced by divergent patterns of gene regulation.
Conflict of interest statement
The authors have declared that no competing interests exist.
Figures
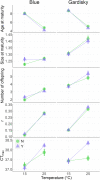
Means ± 1 S.E.M. of age at maturity (days), size at maturity (mm), average number of offspring of the first three clutches, intrinsic growth rate (r), and critical maximum temperature (CTmax, °C) of Daphnia pulicaria collected at Blue and Gardisky Lakes during summer 2017 in response to temperature (15°C and 25°C, x-axis) and predation cues treatments. Circles show response without fish cues (N) and triangles show response to fish cues (Y).
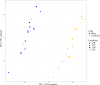
Principal component analysis of gene expression patterns generated through DESeq2 prominently separates individuals by clone along the first principal component.
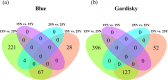
Venn diagram of differentially expressed genes for Daphnia pulicaria clones (a) Blue and (b) Gardisky in response to temperature and predation cues. Cyan and purple sections illustrate differential expression from the introduction of predator cues (Y vs. N for the presence and absence of fish cues), while the green and orange sections illustrate differential expression due to temperature change. Treatment abbreviations: 15Y, 15°C with kairomones; 15N, 15°C without kairomones; 25Y, 25°C with kairomones; 25N, 25°C without kairomones.
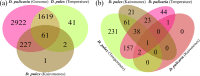
Venn diagrams of orthologous gene clusters between D. pulex DEGs from the Daphnia Stressor Database and (a) the D. pulicaria genome or (b) DEGs from Daphnia pulicaria. Numbers indicate protein families with at least two members, excluding "singletons" that have no orthologous or paralogous matches. Gene identifiers for orthologous clusters and singleton genes are available in (a) S1 File and (b) S2 File.
Similar articles
-
Variation in copper effects on kairomone-mediated responses in Daphnia pulicaria.
DeMille CM, Arnott SE, Pyle GG. DeMille CM, et al. Ecotoxicol Environ Saf. 2016 Apr;126:264-272. doi: 10.1016/j.ecoenv.2015.12.038. Epub 2016 Jan 13. Ecotoxicol Environ Saf. 2016. PMID: 26773836
-
Rapid evolution in response to introduced predators II: the contribution of adaptive plasticity.
Latta LC 4th, Bakelar JW, Knapp RA, Pfrender ME. Latta LC 4th, et al. BMC Evol Biol. 2007 Feb 14;7:21. doi: 10.1186/1471-2148-7-21. BMC Evol Biol. 2007. PMID: 17300732 Free PMC article.
-
Interpopulation variation in a fish predator drives evolutionary divergence in prey in lakes.
Walsh MR, Post DM. Walsh MR, et al. Proc Biol Sci. 2011 Sep 7;278(1718):2628-37. doi: 10.1098/rspb.2010.2634. Epub 2011 Jan 26. Proc Biol Sci. 2011. PMID: 21270045 Free PMC article.
-
He H, Jin H, Jeppesen E, Li K, Liu Z, Zhang Y. He H, et al. Water Res. 2018 Nov 1;144:304-311. doi: 10.1016/j.watres.2018.07.055. Epub 2018 Jul 23. Water Res. 2018. PMID: 30071399
-
Schwarzenberger A, Courts C, von Elert E. Schwarzenberger A, et al. BMC Genomics. 2009 Nov 16;10:527. doi: 10.1186/1471-2164-10-527. BMC Genomics. 2009. PMID: 19917101 Free PMC article.
Cited by
-
Wos G, Palomar G, Marszałek M, Sniegula S. Wos G, et al. Evol Appl. 2024 Sep 6;17(9):e70002. doi: 10.1111/eva.70002. eCollection 2024 Sep. Evol Appl. 2024. PMID: 39247089 Free PMC article.
References
-
- Parmesan C. Ecological and Evolutionary Responses to Recent Climate Change. Annual Review of Ecology, Evolution, and Systematics. 2006;37: 637–669. doi: 10.1146/annurev.ecolsys.37.091305.110100 - DOI