The Medicinal Chemistry of Artificial Nucleic Acids and Therapeutic Oligonucleotides - PubMed
- ️Sat Jan 01 2022
Review
The Medicinal Chemistry of Artificial Nucleic Acids and Therapeutic Oligonucleotides
Miklós Bege et al. Pharmaceuticals (Basel). 2022.
Abstract
Nucleic acids play a central role in human biology, making them suitable and attractive tools for therapeutic applications. While conventional drugs generally target proteins and induce transient therapeutic effects, nucleic acid medicines can achieve long-lasting or curative effects by targeting the genetic bases of diseases. However, native oligonucleotides are characterized by low in vivo stability due to nuclease sensitivity and unfavourable physicochemical properties due to their polyanionic nature, which are obstacles to their therapeutic use. A myriad of synthetic oligonucleotides have been prepared in the last few decades and it has been shown that proper chemical modifications to either the nucleobase, the ribofuranose unit or the phosphate backbone can protect the nucleic acids from degradation, enable efficient cellular uptake and target localization ensuring the efficiency of the oligonucleotide-based therapy. In this review, we present a summary of structure and properties of artificial nucleic acids containing nucleobase, sugar or backbone modifications, and provide an overview of the structure and mechanism of action of approved oligonucleotide drugs including gene silencing agents, aptamers and mRNA vaccines.
Keywords: RNA interference; antisense; aptamer; gene silencing; mRNA vaccine; oligonucleotides; phosphorodiamidate morpholino oligomers (PMOs); phosphorothioate; splicing modulation.
Conflict of interest statement
The authors declare no conflict of interest.
Figures
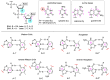
The structure of RNA and DNA and Watson–Crick and Hoogsteen base pair systems.
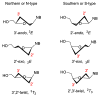
Sugar puckering in nucleic acids.
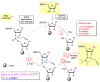
Solid-phase synthesis of oligonucleotides by phosphoramidite chemistry. The iterative steps of synthetic cycle are highlighted in red. DMTr: 4,4′-dimethoxytrityl, TBDMS: t-butyldimethylsilyl, TCA: trichloroacetic acid, Ac2O: acetic anhydride, CPG: controlled pore glass.
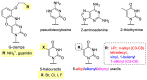
Modified bases in XNAs.
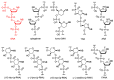
The structure of DNA and XNAs with other sugars than β-D-ribofuranose.
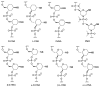
Structure of CNAs, CeNA, HNAs and PMO. PMOs contain both sugar and backbone modifications.
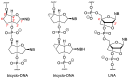
Bi- and tricyclic XNAs.
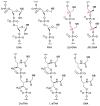
Acyclic NA analogues.
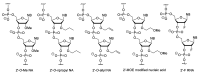
2′-Substituted nucleic acid analogues.
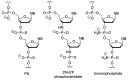
XNAs with negatively charged backbone.
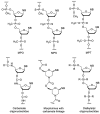
XNAs with neutral backbone.
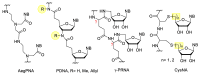
XNAs with peptide type backbones.
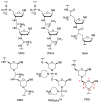
XNAs with positively charged backbone.
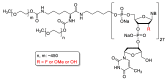
Structure of pegaptanib.
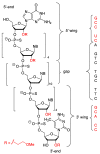
Structure of mipomersen.
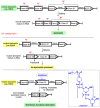
Mechanism of action of eteplirsen.
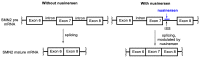
Mechanism of action of nusinersen in patient with SMA. SMA: spinal muscular atrophy, SMN2: survival motor neuron protein gene 2.
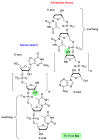
Structure of patisiran.
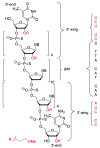
Structure of inotersen.
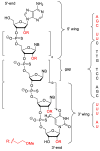
Structure of volanesorsen.
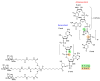
Structure of the GalNAc-siRNA conjugate givosiran.
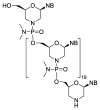
Structure of viltolarsen.
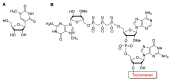
Structure of N1-methylpseudouridine (A) and the 5′-cap of tozinameran (B).
Similar articles
-
Re-Engineering RNA Molecules into Therapeutic Agents.
Egli M, Manoharan M. Egli M, et al. Acc Chem Res. 2019 Apr 16;52(4):1036-1047. doi: 10.1021/acs.accounts.8b00650. Epub 2019 Mar 26. Acc Chem Res. 2019. PMID: 30912917
-
Flynn LL, Li R, Pitout IL, Aung-Htut MT, Larcher LM, Cooper JAL, Greer KL, Hubbard A, Griffiths L, Bond CS, Wilton SD, Fox AH, Fletcher S. Flynn LL, et al. Front Genet. 2022 Apr 6;13:791416. doi: 10.3389/fgene.2022.791416. eCollection 2022. Front Genet. 2022. PMID: 35464859 Free PMC article.
-
Recent progress in non-native nucleic acid modifications.
McKenzie LK, El-Khoury R, Thorpe JD, Damha MJ, Hollenstein M. McKenzie LK, et al. Chem Soc Rev. 2021 Apr 26;50(8):5126-5164. doi: 10.1039/d0cs01430c. Chem Soc Rev. 2021. PMID: 33644787 Review.
-
Liver as a target for oligonucleotide therapeutics.
Sehgal A, Vaishnaw A, Fitzgerald K. Sehgal A, et al. J Hepatol. 2013 Dec;59(6):1354-9. doi: 10.1016/j.jhep.2013.05.045. Epub 2013 Jun 12. J Hepatol. 2013. PMID: 23770039
-
Development of nucleic acid medicines based on chemical technology.
Kawamoto Y, Wu Y, Takahashi Y, Takakura Y. Kawamoto Y, et al. Adv Drug Deliv Rev. 2023 Aug;199:114872. doi: 10.1016/j.addr.2023.114872. Epub 2023 May 25. Adv Drug Deliv Rev. 2023. PMID: 37244354 Review.
Cited by
-
Saffie-Siebert S, Torabi-Pour N, Gibson A, Sutera FM, Dehsorkhi A, Baran-Rachwalska P, Quinn S. Saffie-Siebert S, et al. Mol Ther Methods Clin Dev. 2024 Jul 17;32(3):101299. doi: 10.1016/j.omtm.2024.101299. eCollection 2024 Sep 12. Mol Ther Methods Clin Dev. 2024. PMID: 39239259 Free PMC article.
-
Therapeutic Antisense Oligonucleotides in Oncology: From Bench to Bedside.
Çakan E, Lara OD, Szymanowska A, Bayraktar E, Chavez-Reyes A, Lopez-Berestein G, Amero P, Rodriguez-Aguayo C. Çakan E, et al. Cancers (Basel). 2024 Aug 23;16(17):2940. doi: 10.3390/cancers16172940. Cancers (Basel). 2024. PMID: 39272802 Free PMC article. Review.
-
Current status and trends in small nucleic acid drug development: Leading the future.
Miao Y, Fu C, Yu Z, Yu L, Tang Y, Wei M. Miao Y, et al. Acta Pharm Sin B. 2024 Sep;14(9):3802-3817. doi: 10.1016/j.apsb.2024.05.008. Epub 2024 May 15. Acta Pharm Sin B. 2024. PMID: 39309508 Free PMC article. Review.
-
RNA therapeutics in targeting G protein-coupled receptors: Recent advances and challenges.
Yuan W, Shi X, Lee LTO. Yuan W, et al. Mol Ther Nucleic Acids. 2024 Apr 24;35(2):102195. doi: 10.1016/j.omtn.2024.102195. eCollection 2024 Jun 11. Mol Ther Nucleic Acids. 2024. PMID: 38741614 Free PMC article. Review.
-
Aptamer-Based Strategies to Boost Immunotherapy in TNBC.
Agnello L, d'Argenio A, Nilo R, Fedele M, Camorani S, Cerchia L. Agnello L, et al. Cancers (Basel). 2023 Mar 28;15(7):2010. doi: 10.3390/cancers15072010. Cancers (Basel). 2023. PMID: 37046670 Free PMC article. Review.
References
-
- Carter G.T., McDonald L.A. Uridyl Peptide Antibiotics: Developments in Biosynthesis and Medicinal Chemistry. In: Marinelli F., Genniloud O., editors. Antimicrobials New and Old Molecules in the Fight against Multi-Resistant Bacteria. Springer; Berlin/Heidelberg, Germany: 2014. pp. 177–191.
-
- Rafels-Ybern Á., Torres A.G., Camacho N., Herencia-Ropero A., Frigolé H.R., Wulff T.F., Raboteg M., Bordons A., Grau-Bove X., Ruiz-Trillo I., et al. The expansion of Inosine at the wobble position of tRNAs, and its role in the evolution of proteomes. Mol. Biol. Evol. 2019;36:650–662. doi: 10.1093/molbev/msy245. - DOI - PubMed
Publication types
LinkOut - more resources
Full Text Sources
Other Literature Sources