Exploring the Surface of the Ectodomain of the PD-L1 Immune Checkpoint with Small-Molecule Fragments - PubMed
- ️Sat Jan 01 2022
Exploring the Surface of the Ectodomain of the PD-L1 Immune Checkpoint with Small-Molecule Fragments
Radoslaw Kitel et al. ACS Chem Biol. 2022.
Abstract
Development of small molecules targeting the PD-L1/PD-1 interface is advancing both in industry and academia, but only a few have reached early-stage clinical trials. Here, we take a closer look at the general druggability of PD-L1 using in silico hot spot mapping and nuclear magnetic resonance (NMR)-based characterization. We found that the conformational elasticity of the PD-L1 surface strongly influences the formation of hot spots. We deconstructed several generations of known inhibitors into fragments and examined their binding properties using differential scanning fluorimetry (DSF) and protein-based nuclear magnetic resonance (NMR). These biophysical analyses showed that not all fragments bind to the PD-L1 ectodomain despite having the biphenyl scaffold. Although most of the binding fragments induced PD-L1 oligomerization, two compounds, TAH35 and TAH36, retain the monomeric state of proteins upon binding. Additionally, the presence of the entire ectodomain did not affect the binding of the hit compounds and dimerization of PD-L1. The data demonstrated here provide important information on the PD-L1 druggability and the structure-activity relationship of the biphenyl core moiety and therefore may aid in the design of novel inhibitors and focused fragment libraries for PD-L1.
Conflict of interest statement
The authors declare no competing financial interest.
Figures
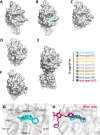
In silico surface probing of PD-L1 with FTMap; (A–F) localization of predicted hot spots on the surface of six X-ray structures of PD-L1, the primary hot spot is represented by probes colored with cyan, (G) architecture of primary hot spots in the BMS-1166-PD-L1 complex, and (H) mapping the main hot spot with the original location of BMS-1166; the hot spot ranking is also shown.
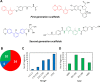
PD-L1 focused fragment library. (A) Structures of known PD-L1 inhibitors used for deconstruction of biphenyl fragments; each subset contains substituted fragments with an overall structure highlighted in red (first generation scaffolds), blue, and green (second generation scaffold). (B) Distribution of fragments in each subset. (C) Distribution of fragments according to calculated M log P values. (D) Distribution of fragments according to molecular weight (MW).
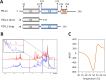
Characteristics of PD-L1 constructs. (A) Domain organization of full-length human PD-L1 (UniProt accession code: Q9NZQ7) and its truncations used in the study. (B) 1H NMR spectra of apo-PD-L1-long (blue) and apo-PD-L1-short (red) with an enlarged aliphatic region. (C) Melting curve of PD-L1-long.
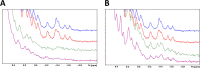
Results of NMR-based validation of selected fragment hits on the PD-L1 ectodomain. (A) Fragments triggering dimerization of PD-L1 ectodomain–1H NMR spectra of apo-PD-L1-long (blue) and with DMSO-d6 (red), TAH4 (green), and TAH47 (purple). (B) Fragments that do not induced PD-L1 ectodomain dimerization–1H NMR spectra of PD-L1-long with TAH35 (green) and STD4 (purple).
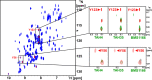
1H–15N HMQC NMR spectra of apo-PD-L1 (blue) and selective labeling of tyrosine in apo-PD-L1 (red) with marked Tyr56 and Tyr123 residues, which form the hydrophobic pocket of PD-L1. In enlarged parts of the spectra, the critical residues’ (Tyr56 and Tyr123) behavior for interactions between PD-L1 and inhibitors is shown (green). In all cases, significant broadening of the signals (disappearance) or their shift is observed under the influence of the tested inhibitors (TAH4 and TAH35) and BMS-1166 as a positive control. For the remaining tyrosine, which is not located in the binding pocket, no signal changes are observed.
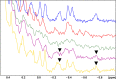
Aliphatic part of 1H NMR spectra of PD-1 (blue), long-PD-L1 (red), the complex of PD-1/long-PD-L1 (green). The complex of PD-1/long-PD-L1 with TAH35 (purple) in the molar ratio protein to the compound 1:20, respectively, and the complex of PD-1/long-PD-L1 with BMS-1166 (as a positive control to show that the complex can be dissociated), the molar ratio 1:1 of the protein and the compound (yellow). Arrows indicate restoring of PD-1 signals.
Similar articles
-
Sasmal P, Kumar Babasahib S, Prashantha Kumar BR, Manjunathaiah Raghavendra N. Sasmal P, et al. Bioorg Med Chem. 2022 Nov 1;73:117001. doi: 10.1016/j.bmc.2022.117001. Epub 2022 Sep 13. Bioorg Med Chem. 2022. PMID: 36126447 Review.
-
Lim H, Chun J, Jin X, Kim J, Yoon J, No KT. Lim H, et al. Sci Rep. 2019 Nov 13;9(1):16727. doi: 10.1038/s41598-019-53216-z. Sci Rep. 2019. PMID: 31723178 Free PMC article.
-
Structural basis for small molecule targeting of the programmed death ligand 1 (PD-L1).
Zak KM, Grudnik P, Guzik K, Zieba BJ, Musielak B, Dömling A, Dubin G, Holak TA. Zak KM, et al. Oncotarget. 2016 May 24;7(21):30323-35. doi: 10.18632/oncotarget.8730. Oncotarget. 2016. PMID: 27083005 Free PMC article.
-
Muszak D, Surmiak E, Plewka J, Magiera-Mularz K, Kocik-Krol J, Musielak B, Sala D, Kitel R, Stec M, Weglarczyk K, Siedlar M, Dömling A, Skalniak L, Holak TA. Muszak D, et al. J Med Chem. 2021 Aug 12;64(15):11614-11636. doi: 10.1021/acs.jmedchem.1c00957. Epub 2021 Jul 27. J Med Chem. 2021. PMID: 34313116 Free PMC article.
-
Chen R, Yuan D, Ma J. Chen R, et al. Future Med Chem. 2022 Jan;14(2):97-113. doi: 10.4155/fmc-2021-0256. Epub 2021 Dec 6. Future Med Chem. 2022. PMID: 34870447 Review.
Cited by
-
Guo Y, Guo Y, Guo Z, Liu B, Xu J. Guo Y, et al. Molecules. 2023 Nov 30;28(23):7881. doi: 10.3390/molecules28237881. Molecules. 2023. PMID: 38067610 Free PMC article.
-
Krutzek F, Donat CK, Ullrich M, Zarschler K, Ludik MC, Feldmann A, Loureiro LR, Kopka K, Stadlbauer S. Krutzek F, et al. Cancers (Basel). 2023 May 6;15(9):2638. doi: 10.3390/cancers15092638. Cancers (Basel). 2023. PMID: 37174103 Free PMC article.
-
Klimek J, Kruc O, Ceklarz J, Kamińska B, Musielak B, van der Straat R, Dӧmling A, Holak TA, Muszak D, Kalinowska-Tłuścik J, Skalniak Ł, Surmiak E. Klimek J, et al. Molecules. 2024 Jun 4;29(11):2646. doi: 10.3390/molecules29112646. Molecules. 2024. PMID: 38893521 Free PMC article.
-
Computer- and NMR-Aided Design of Small-Molecule Inhibitors of the Hub1 Protein.
Reyes Romero A, Kubica K, Kitel R, Rodríguez I, Magiera-Mularz K, Dömling A, Holak TA, Surmiak E. Reyes Romero A, et al. Molecules. 2022 Nov 28;27(23):8282. doi: 10.3390/molecules27238282. Molecules. 2022. PMID: 36500376 Free PMC article.
-
Hec-Gałązka A, Tyrcha U, Barczyński J, Bielski P, Mikitiuk M, Gudz GP, Kitel R, Musielak B, Plewka J, Sitar T, Holak TA. Hec-Gałązka A, et al. ACS Med Chem Lett. 2024 Jun 3;15(6):828-836. doi: 10.1021/acsmedchemlett.4c00042. eCollection 2024 Jun 13. ACS Med Chem Lett. 2024. PMID: 38894909 Free PMC article.
References
-
- Guzik K.; Tomala M.; Muszak D.; Konieczny M.; Hec A.; Błaszkiewicz U.; Pustuła M.; Butera R.; Dömling A.; Holak T. A. Development of the Inhibitors That Target the PD-1/PD-L1 Interaction—A Brief Look at Progress on Small Molecules, Peptides and Macrocycles. Molecules 2019, 24, 2071.10.3390/molecules24112071. - DOI - PMC - PubMed
Publication types
MeSH terms
Substances
LinkOut - more resources
Full Text Sources
Research Materials