Lipid-based nucleic acid therapeutics with in vivo efficacy - PubMed
Review
Lipid-based nucleic acid therapeutics with in vivo efficacy
Md Abu Sufian et al. Wiley Interdiscip Rev Nanomed Nanobiotechnol. 2023 Mar.
Abstract
Synthetic vectors for therapeutic nucleic acid delivery are currently competing significantly with their viral counter parts due to their reduced immunogenicity, large payload capacity, and ease of manufacture under GMP-compliant norms. The approval of Onpattro, a lipid-based siRNA therapeutic, and the proven clinical success of two lipid-based COVID-19 vaccines from Pfizer-BioNTech, and Moderna heralded the specific advantages of lipid-based systems among all other synthetic nucleic acid carriers. Lipid-based systems with diverse payloads-plasmid DNA (pDNA), antisense oligonucleotide (ASO), small interfering RNA (siRNA), microRNA (miRNA), small activating RNA (saRNA), and messenger RNA (mRNA)-are now becoming a mature technology, with growing impact in the clinic. Research over four decades identified the key factors determining the therapeutic success of these multi-component systems. Here, we discuss the main nucleic acid-based technologies, presenting their mechanism of action, delivery barriers facing them, the structural properties of the payload as well as the component lipids that regulate physicochemical properties, pharmacokinetics and biodistribution, efficacy, and toxicity of the resultant nanoparticles. We further detail on the formulation parameters, evolution of the manufacturing techniques that generate reproducible and scalable outputs, and key manufacturing aspects that enable control over physicochemical properties of the resultant particles. Preclinical applications of some of these formulations that were successfully translated from in vitro studies to animal models are subsequently discussed. Finally, clinical success and failure of these systems starting from 1993 to present are highlighted, in a holistic literature review focused on lipid-based nucleic acid delivery systems. This article is categorized under: Therapeutic Approaches and Drug Discovery > Emerging Technologies Therapeutic Approaches and Drug Discovery > Nanomedicine for Oncologic Disease Toxicology and Regulatory Issues in Nanomedicine > Toxicology of Nanomaterials.
Keywords: lipids; lipoplex; nanoparticle; nucleic acid delivery; therapy.
© 2022 Wiley Periodicals LLC.
Conflict of interest statement
Conflict of interest: The authors have no conflict of interest to declare.
Figures
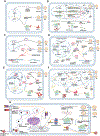
Mechanisms of action of exogenous nucleic acids. Delivery of (A) plasmid and IVT mRNA for protein replacement; (B) antisense oligonucleotides (ASOs) either steric block ASOs or RNAse H competent ASOs. In the nucleus, they can interfere with RNA maturation, whereas in the cytoplasm they can interfere with protein translation; (C) siRNA and shRNA. Exogenous siRNAs can be delivered directly (synthetic siRNAs) or as plasmids encoding shRNA precursors; (D) miRNA mimics and anti-miRs. miRNA mimics replace downregulated endogenous miRNAs whereas anti-miRs inhibit upregulated endogenous miRNAs; (E) saRNA. exogenous saRNAs bind to promoter regions of DNA and increase protein expression by transcriptional activation; (F) CRISPR/Cas9 systems. An RNA-guided (gRNA) Cas9 can introduce double strand DNA break to a target gene. Alternatively, a nuclease deficient Cas9 (dCas9) can either act as a transcriptional activator or as a transcriptional inhibitor; and (G) SAM and NRM mRNA vaccines. mRNA encoding self-amplifying or non-replicating vaccines are processed by endogenous machinery to the viral or other antigens of interest, which are subsequently presented on the cell surface by major histocompatibility complex (MHC) molecules to induce antigen specific responses. This figure was prepared using BioRender (
https://biorender.com/.)
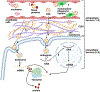
Barriers to nucleic acid delivery. (1) payload degradation by endo- and exo-nucleases; (2) Adsorption of blood proteins or complement on LNP surface with alteration of physicochemical properties of the LNPs; (3) uptake of LNPs by mononuclear phagocyte system; (4) extravasation through endothelial cells; (5) physical barrier of extracellular matrix; (6) uptake of LNP by target cells; (7) escape from endosome; and (8) transport through nuclear envelope (in case of DNA and CRISPR therapeutics). This figure was prepared using BioRender (
https://biorender.com/.)
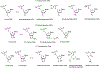
ASO generations and their specific chemistry
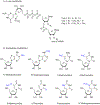
Chemical base modifications frequently used in synthetic mRNA.
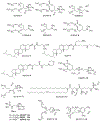
Structurally diverse cationic lipids used to deliver pDNA in vivo
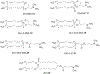
Second generation ionizable lipids with high efficiency in vivo
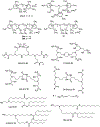
Lipophilic polycations and lipidoids used in LNPs
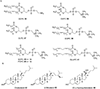
Co-lipids used in LNP formulations: phospholipids (a) and cholesterol derivatives (b)
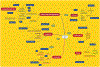
Structural properties and formulation parameters correlated with scLNP physicochemical properties and in vivo performance. This figure was generated in
mindomo.com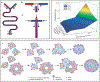
Schematic illustration of microfluidic mixing using A) staggered herringbone mixer, B) in-line T-junction mixer, and C) microfluidic hydrodynamic mixer (top left panel) (Evers et al., 2018); Relationship between total flow rate ratio and siRNA concentration with polydispersity index (PDI) (top right panel) (Belliveau et al., 2012); Mechanism of formation and structure of scLNP in absence (A) and presence (B) of payload (bottom panel) (Kulkarni, Darjuan, et al., 2018).
Similar articles
-
The 60-year evolution of lipid nanoparticles for nucleic acid delivery.
Cullis PR, Felgner PL. Cullis PR, et al. Nat Rev Drug Discov. 2024 Sep;23(9):709-722. doi: 10.1038/s41573-024-00977-6. Epub 2024 Jul 4. Nat Rev Drug Discov. 2024. PMID: 38965378 Review.
-
Structure and Function of Cationic and Ionizable Lipids for Nucleic Acid Delivery.
Sun D, Lu ZR. Sun D, et al. Pharm Res. 2023 Jan;40(1):27-46. doi: 10.1007/s11095-022-03460-2. Epub 2023 Jan 4. Pharm Res. 2023. PMID: 36600047 Free PMC article. Review.
-
Li J, Foged C. Li J, et al. Expert Opin Drug Deliv. 2024 Oct;21(10):1441-1461. doi: 10.1080/17425247.2024.2409142. Epub 2024 Oct 16. Expert Opin Drug Deliv. 2024. PMID: 39387233 Review.
-
Developing Biodegradable Lipid Nanoparticles for Intracellular mRNA Delivery and Genome Editing.
Qiu M, Li Y, Bloomer H, Xu Q. Qiu M, et al. Acc Chem Res. 2021 Nov 2;54(21):4001-4011. doi: 10.1021/acs.accounts.1c00500. Epub 2021 Oct 20. Acc Chem Res. 2021. PMID: 34668716 Review.
-
The role of lipid components in lipid nanoparticles for vaccines and gene therapy.
Hald Albertsen C, Kulkarni JA, Witzigmann D, Lind M, Petersson K, Simonsen JB. Hald Albertsen C, et al. Adv Drug Deliv Rev. 2022 Sep;188:114416. doi: 10.1016/j.addr.2022.114416. Epub 2022 Jul 3. Adv Drug Deliv Rev. 2022. PMID: 35787388 Free PMC article. Review.
Cited by
-
Cardador CM, Muehlmann LA, Coelho CM, Silva LP, Garay AV, Carvalho AMDS, Bastos IMD, Longo JPF. Cardador CM, et al. Pharmaceutics. 2023 Mar 8;15(3):873. doi: 10.3390/pharmaceutics15030873. Pharmaceutics. 2023. PMID: 36986734 Free PMC article. Review.
-
Mechanisms and research advances in mRNA antibody drug-mediated passive immunotherapy.
Zhao Y, Gan L, Ke D, Chen Q, Fu Y. Zhao Y, et al. J Transl Med. 2023 Oct 4;21(1):693. doi: 10.1186/s12967-023-04553-1. J Transl Med. 2023. PMID: 37794448 Free PMC article. Review.
-
Khan SA, Ilies MA. Khan SA, et al. Int J Mol Sci. 2024 Sep 1;25(17):9517. doi: 10.3390/ijms25179517. Int J Mol Sci. 2024. PMID: 39273463 Free PMC article.
-
Chen Z, Shu J, Hu Y, Mei H. Chen Z, et al. Mol Ther. 2024 Nov 6;32(11):3772-3792. doi: 10.1016/j.ymthe.2024.09.019. Epub 2024 Sep 17. Mol Ther. 2024. PMID: 39295145 Review.
-
Biomaterials-mediated CRISPR/Cas9 delivery: recent challenges and opportunities in gene therapy.
Dubey AK, Mostafavi E. Dubey AK, et al. Front Chem. 2023 Sep 28;11:1259435. doi: 10.3389/fchem.2023.1259435. eCollection 2023. Front Chem. 2023. PMID: 37841202 Free PMC article. Review.
References
-
- Adams D, Suhr OB, Dyck PJ, Litchy WJ, Leahy RG, Chen J, Coelho T (2017). Trial design and rationale for APOLLO, a Phase 3, placebo-controlled study of patisiran in patients with hereditary ATTR amyloidosis with polyneuropathy. BMC Neurol, 17(1), 181. doi:10.1186/s12883-017-0948-5 - DOI - PMC - PubMed
Publication types
MeSH terms
Substances
LinkOut - more resources
Full Text Sources
Medical