In-Depth Characterization of the Clostridioides difficile Phosphoproteome to Identify Ser/Thr Kinase Substrates - PubMed
In-Depth Characterization of the Clostridioides difficile Phosphoproteome to Identify Ser/Thr Kinase Substrates
Transito Garcia-Garcia et al. Mol Cell Proteomics. 2022 Nov.
Abstract
Clostridioides difficile is the leading cause of postantibiotic diarrhea in adults. During infection, the bacterium must rapidly adapt to the host environment by using survival strategies. Protein phosphorylation is a reversible post-translational modification employed ubiquitously for signal transduction and cellular regulation. Hanks-type serine/threonine kinases (STKs) and serine/threonine phosphatases have emerged as important players in bacterial cell signaling and pathogenicity. C. difficile encodes two STKs (PrkC and CD2148) and one phosphatase. We optimized a titanium dioxide phosphopeptide enrichment approach to determine the phosphoproteome of C. difficile. We identified and quantified 2500 proteins representing 63% of the theoretical proteome. To identify STK and serine/threonine phosphatase targets, we then performed comparative large-scale phosphoproteomics of the WT strain and isogenic ΔprkC, CD2148, Δstp, and prkC CD2148 mutants. We detected 635 proteins containing phosphorylated peptides. We showed that PrkC is phosphorylated on multiple sites in vivo and autophosphorylates in vitro. We were unable to detect a phosphorylation for CD2148 in vivo, whereas this kinase was phosphorylated in vitro only in the presence of PrkC. Forty-one phosphoproteins were identified as phosphorylated under the control of CD2148, whereas 114 proteins were phosphorylated under the control of PrkC including 27 phosphoproteins more phosphorylated in the ∆stp mutant. We also observed enrichment for phosphothreonine among the phosphopeptides more phosphorylated in the Δstp mutant. Both kinases targeted pathways required for metabolism, translation, and stress response, whereas cell division and peptidoglycan metabolism were more specifically controlled by PrkC-dependent phosphorylation in agreement with the phenotypes of the ΔprkC mutant. Using a combination of approaches, we confirmed that FtsK was phosphorylated in vivo under the control of PrkC and that Spo0A was a substrate of PrkC in vitro. This study provides a detailed mapping of kinase-substrate relationships in C. difficile, paving the way for the identification of new biomarkers and therapeutic targets.
Keywords: Hanks kinase; cell division; envelope homeostasis; phosphatase; serine phosphorylation; threonine phosphorylation.
Copyright © 2022 The Authors. Published by Elsevier Inc. All rights reserved.
Conflict of interest statement
Conflict of interest The authors declare no competing interests.
Figures
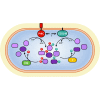
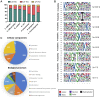
Overview of the Clostridioides difficile 630Δerm phosphoproteome.A, distribution of phosphorylated residues across all phosphoproteins found in C. difficile compared with other Gram-positives (Bacillus subtilis, Streptococcus pneumoniae, Streptococuus pyogenes, and Listeria monocytogenes) and Gram-negative (Escherichia coli) bacteria (14, 20, 50, 77). pS means phosphoserine, pT phosphothreonine, and pY phosphotyrosine. B, significantly enriched motifs in the phosphopeptides detected in the C. difficile WT strain 630Δerm (p < 1e-6). A background of 100,000 random sequences around the S, T, or Y amino acids was used to estimate the motif scores (Sc). The higher the motif scores, the more the motifs are overrepresented against the background. C, Gene Ontology distribution of identified phosphoproteins with respect to biological processes and cellular components with the DAVID functional annotation tools. The percentages indicate the proteins that belong to each category. S, serine; T, threonine; Y, tyrosine.
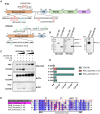
Phosphorylation of STKs in vivo and in vitro.A, a schematic presentation of PrkC and CD2148 containing location of the different domains is indicated. The kinase domain (KD) containing the activation loop, the transmembrane (TM) region, the PASTA domains, and the Ser-Gly-Asn (SGN) rich segment are indicated. Phosphoresidues detected in vivo and in vitro are indicated in red, and phosphoresidues detected only in vivo are indicated in blue (supplemental Table S3) and only in vitro in green. B, in vitro phosphorylation of purified PrkC-KD and CD2148-KD proteins in presence of 5 mM ATP analyzed by SDS-PAGE, Phos-Tag, and Western blot using anti-pT antibodies (⍺-pT). C, in vitro phosphorylation of CD2148-KD in the presence of PrkC-KD analyzed by Western blot using anti-pT (⍺-Thr) and anti-pS (⍺-Ser) antibodies. D, mass spectrometry detection of CD2148 phosphorylation sites after in vitro phosphorylation reactions performed without or with PrkC-KD at ratio 1:1 and 1:10. E, multiple sequence alignment of activation loop of PrkC (pink) and CD2148 (gray) with homologous STKs: Staphylococcus aureus PknB, Bacillus subtilis PrkC, and Enterococcus faecalis IreK. Conserved residues are marked in blue color, and phosphorylated residues are shown in red boxes. The T/S amino acid of the GT/S motif is indicated by a black arrow. KD, kinase domain; PASTA, penicillin-binding and STK-associated; pS, phosphoserine; pT, phosphothreonine; S, serine; STK, serine/threonine kinase; T, threonine.
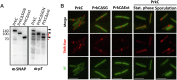
PASTA domains are not necessary for PrkC autophosphorylation and for its septal localization.A, Western blots of whole-cell lysates from cells expressing SNAP–PrkC or truncated versions were probed with anti-SNAP antibodies (⍺-SNAP) and anti-pT antibodies (⍺-pT). The PrkC phosphorylation signal is indicated by a black arrow, and unknown phosphorylated proteins are indicated in red asterisk (∗). B, localization of full-length and truncated SNAP–PrkC. Merge images, TMR-Star fluorescent signal and autofluorescence. The scale bar represents 5 μm. PASTA, penicillin-binding and STK-associated; pT, phosphothreonine.
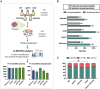
Phosphoproteomic analysis of STKs and STP mutants.A, schematic workflow for quantitative phosphoproteomic analysis of the WT (630Δerm) strain and the ΔprkC, CD2148, Δdouble (ΔprkC CD2148), and Δstp mutants. Five replicates were performed for each strain. Extracted protein samples were digested with trypsin followed by a phosphopeptide TiO2 enrichment using our optimized protocol. The resulting samples were analyzed by nano LC–MS/MS on a Q Exactive HF mass spectrometer, and average number of identified proteins and phosphosites is shown at the bottom. B, number of identified proteins (2493 in total) and phosphoproteins for each strain (for a total number of 1735 identified phosphopeptides, 1513 have an LP > 0.75 corresponding to 635 phosphoproteins). C, distribution of phosphorylation sites across all phosphoproteins found in each strain. pS means phosphoserine, pT phosphothreonine, and pY phosphotyrosine. A significantly different proportion of pT is observed between the Δstp mutant and the WT strain (p < 5%). LP, localization probability; STK, serine/threonine kinase; STP, serine/threonine phosphatase; TiO2, titanium dioxide.
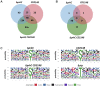
Identified phosphorylation changes in Clostridioides difficile mutants inactivated for the genes encoding STK or STP. Venn diagram showing overlaps of the phosphopeptides (LP > 0.75) significantly less abundant in the kinase mutants (ΔprkC, CD2148, or double) compared with WT strain (A) or also more abundant in the Δstp mutant compared with WT strain (B) (supplemental Table S7). C, phosphorylation site motif analysis highlighting the distribution of amino acids at positions adjacent to the pT or pS in phosphopeptide lists. Phosphopeptides less abundant in the ΔprkC (top left), CD2148 (top right), or the double mutants (bottom left) than in the WT strain. In bottom right, motif analysis of phosphopeptides more abundant in the Δstp mutant among the phosphopeptides less abundant in the ΔprkC, CD2148, or double mutants than in the WT strain. The ggseqlogo R package was used. LP, localization probability; pT, phosphothreonine; pS, phosphoserine; STK, serine/threonine kinase; STP, serine/threonine phosphatase.
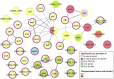
Protein targets of STK and STP in Clostridioides difficile. Protein–protein interaction network of proteins with phosphopeptides less abundant in the STK mutants compared with the WT strain and more abundant in the Δstp mutant compared with the WT strain. STK, serine/threonine kinase; STP, serine/threonine phosphatase.

Phosphorylation of Spo0A by PrkC.A, schematic presentation of the location of the different domains of Spo0A. The phosphorylated S and T residues identified in vivo are indicated in red. The D61 residue phosphorylated by the histidine kinases associated with Spo0A is also indicated. B, in vitro phosphorylation assay of Spo0A by the STKs at different ratio of kinase:substrate. Phosphorylation was visualized by a Phos-Tag acrylamide allowing the separation of phosphorylated and nonphosphorylated proteins. Molecular mass standards are shown on the left. C, mass spectrometry detection of Spo0A phosphorylation sites after in vitro phosphorylation reactions performed without or with PrkC. D, in vitro phosphorylation of the Spo0A-DBD by PrkC. Phosphorylation was visualized by Phos-Tag fluorescent gel stain reagents. E, mass spectrometry detection of Spo0A-DBD phosphorylation sites. DBD, DNA-binding domain; S, serine; STK, serine/threonine kinase; T, threonine.
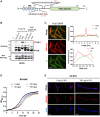
PrkC-dependent phosphorylation of FtsK at residues localized within the flexible linker.A, schematic presentation of the different domains of FtsK. STK/STP-dependent phosphorylated residues detected in vivo are indicated in red color (supplemental Table S3). B, Phos-Tag and non–Phos-Tag SDS-PAGE with the whole-cell lysates of different strains expressing ftsK-HA or ftsKT318A-HA under the control of the Ptet promoter. The FtsK-HA protein was detected using an anti–HA-tag antibody. C, FtsK–SNAP localization. The expression of the PtetftsK–SNAP fusion carried by a plasmid introduced into the WT strain was induced 2 h in the presence of 50 ng/ml of ATc. Merge images, TMR-Star fluorescent signal and autofluorescence (AF) are presented. Quantification of fluorescence in arbitrary units (a.u) along the major and minor axis for a typical cell. The scale bar represents 5 μm. D, growth curves of cells expressing an antisense RNA targeting the initiation of translation of the ftsK gene. E, fluorescence microscopy of cells expressing the ftsK antisense (in the presence of 100 ng/ml of ATc) stained with the membrane dye FM4-64 and DAPI for the chromosome. The scale bar represents 5 μm. ATc, anhydrotetracycline; DAPI, 4′,6-diamidino-2-phenylindole; HA, hemagglutinin; STK, serine/threonine kinase; STP, serine/threonine phosphatase.
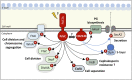
Major targets of Clostridioides difficile STKs involved in cell division and envelope homeostasis. Schematic representation of major phosphorylated proteins involved in cell division and envelope homeostasis identified as STK/STP dependent in this study. Black and blue arrows mean PrkC- and CD2148-dependent phosphorylation, respectively. PrkC could sense extracellular signals generated during PG synthesis (muropeptides, lipid II, or other) through its PASTA domains. PASTA, penicillin-binding and STK-associated; PG, peptidoglycan; STK, serine/threonine kinase; STP, serine/threonine phosphatase.
Similar articles
-
Garcia-Garcia T, Poncet S, Cuenot E, Douché T, Giai Gianetto Q, Peltier J, Courtin P, Chapot-Chartier MP, Matondo M, Dupuy B, Candela T, Martin-Verstraete I. Garcia-Garcia T, et al. mBio. 2021 May 18;12(3):e00519-21. doi: 10.1128/mBio.00519-21. mBio. 2021. PMID: 34006648 Free PMC article.
-
Cuenot E, Garcia-Garcia T, Douche T, Gorgette O, Courtin P, Denis-Quanquin S, Hoys S, Tremblay YDN, Matondo M, Chapot-Chartier MP, Janoir C, Dupuy B, Candela T, Martin-Verstraete I. Cuenot E, et al. Infect Immun. 2019 Jul 23;87(8):e00005-19. doi: 10.1128/IAI.00005-19. Print 2019 Aug. Infect Immun. 2019. PMID: 31085703 Free PMC article.
-
Ravikumar V, Shi L, Krug K, Derouiche A, Jers C, Cousin C, Kobir A, Mijakovic I, Macek B. Ravikumar V, et al. Mol Cell Proteomics. 2014 Aug;13(8):1965-78. doi: 10.1074/mcp.M113.035949. Epub 2014 Jan 5. Mol Cell Proteomics. 2014. PMID: 24390483 Free PMC article.
-
Janczarek M, Vinardell JM, Lipa P, Karaś M. Janczarek M, et al. Int J Mol Sci. 2018 Sep 21;19(10):2872. doi: 10.3390/ijms19102872. Int J Mol Sci. 2018. PMID: 30248937 Free PMC article. Review.
-
Phosphoproteomic Approaches to Discover Novel Substrates of Mycobacterial Ser/Thr Protein Kinases.
Baros SS, Blackburn JM, Soares NC. Baros SS, et al. Mol Cell Proteomics. 2020 Feb;19(2):233-244. doi: 10.1074/mcp.R119.001668. Epub 2019 Dec 15. Mol Cell Proteomics. 2020. PMID: 31839597 Free PMC article. Review.
Cited by
-
Bacterial metabolites influence the autofluorescence of Clostridioides difficile.
Ticer TD, Tingler AM, Glover JS, Dooley SA, Kendrick J, Zackular JP, Devkota S, Wu GD, Mahalak K, Engevik A, Engevik MA. Ticer TD, et al. Front Microbiol. 2024 Oct 8;15:1459795. doi: 10.3389/fmicb.2024.1459795. eCollection 2024. Front Microbiol. 2024. PMID: 39439940 Free PMC article.
-
Mikkat S, Kreutzer M, Patenge N. Mikkat S, et al. Microorganisms. 2024 Mar 20;12(3):621. doi: 10.3390/microorganisms12030621. Microorganisms. 2024. PMID: 38543672 Free PMC article.
-
The multiplicity of thioredoxin systems meets the specific lifestyles of Clostridia.
Anjou C, Lotoux A, Zhukova A, Royer M, Caulat LC, Capuzzo E, Morvan C, Martin-Verstraete I. Anjou C, et al. PLoS Pathog. 2024 Feb 8;20(2):e1012001. doi: 10.1371/journal.ppat.1012001. eCollection 2024 Feb. PLoS Pathog. 2024. PMID: 38330058 Free PMC article.
-
A Review of the Bacterial Phosphoproteomes of Beneficial Microbes.
Lim S. Lim S. Microorganisms. 2023 Apr 3;11(4):931. doi: 10.3390/microorganisms11040931. Microorganisms. 2023. PMID: 37110354 Free PMC article. Review.
-
Miao X, Zhao Y, Li H, Ren Y, Hu G, Yang J, Liu L, Li X. Miao X, et al. Animals (Basel). 2022 Dec 25;13(1):78. doi: 10.3390/ani13010078. Animals (Basel). 2022. PMID: 36611688 Free PMC article.
References
-
- Hanks S.K., Quinn A.M., Hunter T. The protein kinase family: conserved features and deduced phylogeny of the catalytic domains. Science. 1988;241:42–52. - PubMed
-
- Stancik I.A., Šestak M.S., Ji B., Axelson-Fisk M., Franjevic D., Jers C., et al. Serine/threonine protein kinases from bacteria, archaea and eukarya share a common evolutionary origin deeply rooted in the tree of life. J. Mol. Biol. 2018;430:27–32. - PubMed
Publication types
MeSH terms
Substances
LinkOut - more resources
Full Text Sources
Molecular Biology Databases