Interaction of client-the scaffold on which FeS clusters are build-with J-domain protein Hsc20 and its evolving Hsp70 partners - PubMed
- ️Sat Jan 01 2022
Review
Interaction of client-the scaffold on which FeS clusters are build-with J-domain protein Hsc20 and its evolving Hsp70 partners
Jaroslaw Marszalek et al. Front Mol Biosci. 2022.
Abstract
In cells molecular chaperone systems consisting of Hsp70 and its obligatory J-domain protein (JDP) co-chaperones transiently interact with a myriad of client proteins-with JDPs typically recruiting their partner Hsp70 to interact with particular clients. The fundamentals of this cyclical interactions between JDP/Hsp70 systems and clients are well established. Much less is known about other aspects of JDP/Hsp70 system function, including how such systems evolved over time. Here we discuss the JDP/Hsp70 system involved in the biogenesis of iron-sulfur (FeS) clusters. Interaction between the client protein, the scaffold on which clusters are built, and its specialized JDP Hsc20 has stayed constant. However, the system's Hsp70 has changed at least twice. In some species Hsc20's Hsp70 partner interacts only with the scaffold, in others it has many JDP partners in addition to Hsc20 and interacts with many client proteins. Analysis of this switching of Hsp70 partners has provided insight into the insulation of JDP/Hsp70 systems from one another that can occur when more than one Hsp70 is present in a cellular compartment, as well as how competition among JDPs is balanced when an Hsp70 partner is shared amongst a number of JDPs. Of particularly broad relevance, even though the scaffold's interactions with Hsc20 and Hsp70 are functionally critical for the biogenesis of FeS cluster-containing proteins, it is the modulation of the Hsc20-Hsp70 interaction per se that allows Hsc20 to function with such different Hsp70 partners.
Keywords: FeS cluster biogenesis; Hsp70-client interaction cycle; Isu/IscU scaffold; molecular chaperones; post-duplication functional divergence; protein evolution; protein interactions.
Copyright © 2022 Marszalek and Craig.
Conflict of interest statement
The authors declare that the research was conducted in the absence of any commercial or financial relationships that could be construed as a potential conflict of interest.
Figures
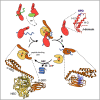
JDP/Hsp70 client binding cycle and structures of key components. (upper left) Different JDPs (red) can deliver different clients, indicated by different colors and shapes, to the same Hsp70 by interacting with them directly via distinct client binding domains, indicated by different shapes. (center) This scheme depicts the cycle involved in folding of a client protein. The same cycle occurs when JDP/Hsp70 systems are involved in other cellular processes, including FeS cluster biogenesis (see Figure 3): (i) JDP-client complex interacts with ATP bound Hsp70. In this “open” conformation the nucleotide binding domain (NBD, beige) is docked on to the substrate binding domain (SBD, brown) and the interdomain flexible linker (black) is bound in a crevice of the NBD. This conformation allows easy access of client to the peptide binding cleft of β-SBD, as α-SBD is retracted from it, and enables binding of the J-domain, which interacts at the NBD-β-SBD interface. It also allows simultaneous, transient interactions of the J-domain and JDP-bound client, and thus synergistic stimulation of ATP hydrolysis by Hsp70. (ii) ATP hydrolysis triggers conformational changes resulting in separation of the NBD and SBD, only connected by a flexible linker. The α-SBD subdomain covers the peptide binding cleft of β-SBD, stabilizing its interaction with the client. Separation of the SBD and NBD also results in loss of the J-domain binding face, and thus JDP release. (iii) ADP exchange for ATP, often involving a nucleotide exchange factor (NEF), reverting Hsp70 to the ATP bound state, causing client release. Structures of critical components of the cycle from E. coli DnaK and DnaJ—the best studied JDP/Hsp70 system—are depicted (bottom left) the ATP bound conformation of DnaK (PDB ID: 4B9Q). (Upper right) J-domain architecture is conserved with helices 2 and 3 (H-2, H-3) forming a finger like structure. Loop between these helices contains His Pro Asp (HPD) sequence present in all J-domains and required for the stimulation of Hsp70s’ ATPase. (bottom right) Hsp70’s SBD interacting with a peptide derived from a client protein (PDB ID: 1DKZ). Note helical α-SBD subdomain covering the binding cleft of β-SBD, but without contacting the client.
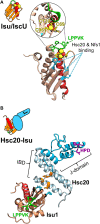
Structural models of the FeS cluster scaffold and its complex with Hsc20. (A) Cartoon depicting key structural elements of FeS cluster loaded (yellow/red balls) Isu/IscU scaffold and homology model of S. cerevisiae Isu1 with bound FeS cluster based on the crystal structure of FeS cluster bound IscU from Aquifex aeolicus (PDB ID: 2Z7E) at left and right, respectively. Insert within the circle is the structure tilted 90° to visualize the three Cys residues (yellow) that coordinate a 2Fe-2S cluster (yellow/red balls). LPPVK sequence (green) localized on a loop prior to C-terminal helix 5 (red) is the binding site for Hsp70. Hot spot residues involved in the Isu1 interactions with both Hsc20 and cysteine desulfurase Nfs1 are indicated (cyan). Note: the Hsc20 and Hsp70 binding sites on Isu1 do not overlap. (B) Cartoon representing J-domain protein Hsc20 in complex with Isu/IscU and structural model of the Hsc20 of S. cerevisiae (PDB ID: 3UO2 and 3UO3) in complex with Isu1 at left and right, respectively. Hsc20 is a simple JDP consisting of the N-terminal J-domain (dark cyan) with HPD motif (magenta) and C-terminal Isu binding domain, IBD (light cyan), which interacts with Isu via a contact surface involving hot spot residues (orange).
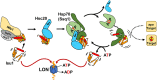
The role of Hsc20/Hsp70 scaffold binding cycle in FeS cluster biogenesis. Interaction of the Isu/IscU scaffold, which is structurally highly dynamic (indicated by agitrons) with a FeS cluster assembly complex (Nfs1/Isd11/Acp1 and other accessory proteins: frataxin, ferredoxin-not shown) is required for de novo cluster synthesis. The cluster loaded scaffold interacts with the dedicated JDP Hsc20. The scaffold interactions with both the cluster assembly complex and Hsc20 protect it from degradation by LON protease. Hsc20-scaffold complex interacts with its Hsp70 partner in the ATP bound conformation. Synergistic stimulation of Hsp70’s ATPase by the J-domain of Hsc20 and the scaffold triggers conformational changes that leads to Hsc20 release and stabilization of an Hsp70-scaffold complex. Formation of this complex is required for effective cluster transfer onto apo-target proteins. Exchange of ADP for ATP results in scaffold release. It is not clear what regulates whether a scaffold is reused in another round of cluster assembly/transfer or proteolyzed.
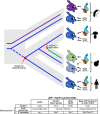
Hsc20 has changed Hsp70 partners twice across the tree of life. (top) Gray contour indicates phylogenetic relationships among evolving lineages: bacteria, fungi, metazoa. Solid lines indicate evolution of Hsp70s HscA (magenta) and DnaK (blue); broken blue line indicates the eukaryotic lineage prior to the origin of mitochondria (red dot). JDP Hsc20 is found in bacteria and mitochondria of eukaryotes (cyan, shown bound to client Isu/IscU in brown with FeS cluster). Solid arrows indicate its Hsp70 partner. In bacteria Hsc20 (HscB) partners with FeS cluster specialized Hsp70 HscA (magenta). This system coexists with multifunctional Hsp70 DnaK (dark blue). In mitochondria of eukaryotic cells Hsc20 partners with mtHsp70 e.g., most fungi and metazoans (indicated by mushroom and human pictograms) or with FeS cluster specialized Hsp70 Ssq1 (green), which evolved after an Hsp70 gene duplication (red arrow) in a progenitor of the S. cerevisiae lineage (budding yeast pictogram). The other duplicate, Ssc1 (light blue) remained multifunctional partnering with multiple JDPs, but only minimally with Hsc20, as indicated by dotted line. Following the origin of mitochondria Hsc20 was maintained, but HscA was not. Its function in FeS cluster biogenesis was substituted by mtHsp70, a descendant of DnaK (dark blue). (bottom) Names of JDPs and Hsp70s functioning in FeS cluster biogenesis. Those in parentheses are the name of human proteins.
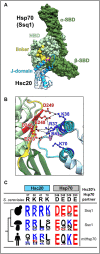
J-domain-Hsp70 interaction involves evolutionary variable interface. (A) Structural model of the S. cerevisiae Hsc20-Ssq1 (ATP) complex (Tomiczek et al., 2020). As in all JDP-Hsp70 interactions, the J-domain binds at the interface of NBD, β-SBD and interdomain linker formed when Ssq1 is in the ATP-bound conformation. Hsc20 in cyan with J-domain dark, Isu binding domain, light; HPD motif (magenta). Ssq1: NBD (light green), α- and β-SBDs (dark green); interdomain linker (yellow) is bound to the NBD. (B) The interconnected network of contacts between positively charged residues of helix 2 of the J-domain (blue) and negatively charged residues of Ssq1 (red) is critical for J-domain interaction with Ssq1; electrostatic interactions are depicted as broken lines (black). Note that one residue on the J-domain side interacts with more than one residue on the Hsp70 side e.g., J-domain’s R41 contacts D246 and E253 of Ssq1. K70 of helix 3 is also critical for Hsc20-Ssq1 complex formation, though its binding partner(s) was not identified. (C) The key residues involved in the J-domain-Ssq1 interaction are evolutionary variable. Sequence logos represent the amino acid frequency at positions of Hsc20 and Hsp70, which are homologous to the hot spot positions of the S. cerevisiae Hsc20 J-domain-Ssq1 interface; positively charged (blue), negatively charged (red) and uncharged (black). The analysis was based on Hsc20 and Hsp70 sequences from 23 yeast species, which diverged after Hsp70 gene duplication leading to Ssq1, 47 pre-duplication fungi species (harboring only mtHsp70) and 20 metazoan species (harboring mtHsp70), designated by human figure. Note: in contrast to the invariant HPD motif hot spot residues involved in J-domain/Ssq1 interaction in S. cerevisiae are evolutionary variable, particularly outside the post-duplication species.
Similar articles
-
Jelen M, Grochowina I, Grabinska-Rogala A, Ciesielski SJ, Dabrowska K, Tomiczek B, Nierzwicki L, Delewski W, Schilke B, Czub J, Dadlez M, Dutkiewicz R, Craig EA, Marszalek J. Jelen M, et al. J Mol Biol. 2023 Nov 1;435(21):168283. doi: 10.1016/j.jmb.2023.168283. Epub 2023 Sep 18. J Mol Biol. 2023. PMID: 37730084 Free PMC article.
-
Biochemical Convergence of Mitochondrial Hsp70 System Specialized in Iron-Sulfur Cluster Biogenesis.
Kleczewska M, Grabinska A, Jelen M, Stolarska M, Schilke B, Marszalek J, Craig EA, Dutkiewicz R. Kleczewska M, et al. Int J Mol Sci. 2020 May 8;21(9):3326. doi: 10.3390/ijms21093326. Int J Mol Sci. 2020. PMID: 32397253 Free PMC article.
-
Chaperone function in Fe-S protein biogenesis: Three possible scenarios.
Marszalek J, Craig EA, Pitek M, Dutkiewicz R. Marszalek J, et al. Biochim Biophys Acta Mol Cell Res. 2024 Jun;1871(5):119717. doi: 10.1016/j.bbamcr.2024.119717. Epub 2024 Apr 2. Biochim Biophys Acta Mol Cell Res. 2024. PMID: 38574821 Review.
-
Marszalek J, Craig EA, Tomiczek B. Marszalek J, et al. Subcell Biochem. 2023;101:293-318. doi: 10.1007/978-3-031-14740-1_10. Subcell Biochem. 2023. PMID: 36520311
-
Molecular chaperones HscA/Ssq1 and HscB/Jac1 and their roles in iron-sulfur protein maturation.
Vickery LE, Cupp-Vickery JR. Vickery LE, et al. Crit Rev Biochem Mol Biol. 2007 Mar-Apr;42(2):95-111. doi: 10.1080/10409230701322298. Crit Rev Biochem Mol Biol. 2007. PMID: 17453917 Review.
Cited by
-
The roles of molecular chaperones in regulating cell metabolism.
Binder MJ, Pedley AM. Binder MJ, et al. FEBS Lett. 2023 Jul;597(13):1681-1701. doi: 10.1002/1873-3468.14682. Epub 2023 Jun 16. FEBS Lett. 2023. PMID: 37287189 Free PMC article. Review.
-
Jelen M, Grochowina I, Grabinska-Rogala A, Ciesielski SJ, Dabrowska K, Tomiczek B, Nierzwicki L, Delewski W, Schilke B, Czub J, Dadlez M, Dutkiewicz R, Craig EA, Marszalek J. Jelen M, et al. J Mol Biol. 2023 Nov 1;435(21):168283. doi: 10.1016/j.jmb.2023.168283. Epub 2023 Sep 18. J Mol Biol. 2023. PMID: 37730084 Free PMC article.
References
-
- Adrover M., Howes B. D., Iannuzzi C., Smulevich G., Pastore A. (2015). Anatomy of an iron-sulfur cluster scaffold protein: Understanding the determinants of [2Fe-2S] cluster stability on IscU. Biochimica Biophysica Acta (BBA) - Mol. Cell Res. 1853 (6), 1448–1456. 10.1016/j.bbamcr.2014.10.023 - DOI - PubMed
Publication types
LinkOut - more resources
Full Text Sources
Miscellaneous