Promotion of diet-induced obesity and metabolic syndromes by BID is associated with gut microbiota - PubMed
. 2022 Dec;6(12):3349-3362.
doi: 10.1002/hep4.2052. Epub 2022 Nov 15.
Affiliations
- PMID: 36382356
- PMCID: PMC9701492
- DOI: 10.1002/hep4.2052
Promotion of diet-induced obesity and metabolic syndromes by BID is associated with gut microbiota
Shengmin Yan et al. Hepatol Commun. 2022 Dec.
Abstract
A growing body of evidence has indicated an expanding functional network of B-cell lymphoma 2 (BCL-2) family proteins beyond regulation of cell death and survival. Here, we examined the role and mechanisms of BH3 interacting-domain death agonist (BID), a pro-death BCL-2 family member, in the development of diet-induced metabolic dysfunction. Mice deficient in bid (bid-/- ) were resistant to high-fat diet (HFD)-induced obesity, hepatic steatosis, and dyslipidemia with an increased insulin sensitivity. Indirect calorimetry analysis indicated that bid deficiency increased metabolic rate and decreased respiratory exchange ratio, suggesting a larger contribution of lipids to overall energy expenditure. While expression of several genes related to lipid accumulation was only increased in wild-type livers, metabolomics analysis revealed a consistent reduction in fatty acids but an increase in certain sugars and Krebs cycle intermediates in bid-/- livers. Gut microbiota (GM) analysis indicated that HFD induced gut dysbiosis with differential patterns in wild-type and in bid-/- mice. Notably, abrogation of GM by antibiotics during HFD feeding eliminated the beneficial effects against obesity and hepatic steatosis conferred by the bid deficiency. Conclusion: These results indicate that the protective role of bid-deficiency against diet-induced metabolic dysfunction interacts with the function of GM.
© 2022 The Authors. Hepatology Communications published by Wiley Periodicals LLC on behalf of American Association for the Study of Liver Diseases.
Conflict of interest statement
Nothing to report.
Figures
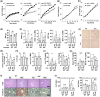
Mice that are deficient in BH3 interacting‐domain death agonist (bid −/− ) are resistant to high‐fat diet (HFD)–induced obesity, hepatic steatosis, dyslipidemia, and liver injury. (A) Male wild‐type (WT) and bid‐deficient (bid −/− ) mice were fed with regular chow diet (RD), HFD, or high‐fat high‐carbohydrate diet (HFHCD) for 16 weeks (n = 5–8). The body weight (BW) was measured every 2 weeks. (B) Female WT and bid −/− mice were fed with HFD for 24 weeks (n = 5–8). The body weight was measured every 2 weeks. (C) Male mice with the genotypes of Ob/Ob or bid −/− :Ob/Ob were monitored for the body weight at different ages under RD (n = 5–8). (D) Male WT and bid −/− mice were fed with RD or HFD for 10 weeks. The body weight was measured at the end of 10 weeks. (E) Weight and the percentage of the body weight of epididymal white adipose tissue (eWAT) and interscapular brown adipose tissue (iBAT) in HFD‐fed WT and bid −/− mice. (F) Hematoxylin and eosin (H&E) staining of eWAT from mice with indicated genotypes and diet (×200). (G). Relative adipocyte size of eWAT with fold change over the WT‐RD group. (H) Liver weight and percentage of liver weight over body weight were measured in male mice with designated genotypes and given the indicated diets for 10 weeks. (I) Representative images of hepatic H&E staining (×100) and Oil Red O staining (×200). (J,K) Triglyceride (TG) and total cholesterol (TC) levels in the liver (C) and the serum (D) (n = 3–5). (L) Serum levels of alanine aminotransferase (ALT) and aspartate aminotransferase (AST) in male mice given the indicated diet for 10 or 20 weeks. Data are shown as means ± SEM. Data are shown as means ± SEM. Groups with different letters or asterisk had significant differences (p < 0.05). N.S., no statistical significance.
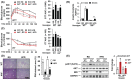
bid −/− mice demonstrate higher sensitivity to insulin than WT mice. Male WT and bid‐deficient (bid −/− ) mice on RD or HFD for 10 weeks were subjected to the following assays: glucose tolerance test (GTT) (A), measurement of serum levels of insulin before and after glucose challenge (B), insulin tolerance test (ITT) (C), measurement of hepatic glycogen levels with periodic acid–Schiff (PAS) staining (D), and phosphorylation of hepatic protein kinase B (AKT) (E) following insulin challenge. For (A) and (C), n = 4–5. For (D), percentage of positive area was quantified with Image‐J (n = 3). For (E), phosphorylation levels of AKT were calculated as the ratio of phosphorylated and total protein levels (phos/total) and expressed as fold change of WT mice with RD group (n = 3–4). Data were shown as means ± SEM. Groups with different letters or asterisks (B) had significant differences (p < 0.05). AUC, area under curve; GAPDH, glyceraldehyde 3‐phosphate dehydrogenase.
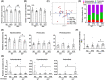
Alteration of gut microbiota (GM) in bid −/− mice after HFD feeding at the phylum level. Male WT and bid‐deficient (bid −/− ) mice were on RD or HFD for 10 weeks. (A,B) Shannon species diversity (A) and the number of identified S16 species (B) were determined in post‐experiment fecal samples. (C) Principal coordinates analysis (PCoA) based on relative abundance at species level shows a distinguishable profile of GM between HFD and RD mice. (D,E) Proportion of dominant bacteria (D) and relative abundance of major bacteria (E) at the phylum level in post‐experiment fecal samples. (F) Ratio of Firmicutes and Bacteroidetes in post‐experiment fecal samples. (G) Proportion of Actinobacteria, Cyanobacteria, and Chloroflexi was higher in HFD‐fed bid −/− mice compared with HFD‐fed WT mice. Data are shown as median with interquartile range, n = 10/group. Mann–Whitney test; *p < 0.05.
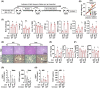
Resistance of bid −/− mice to diet‐induced obesity (DIO) and hepatic steatosis is eliminated with antibiotics (ABX) treatment. (A) Scheme of HFD feeding in combination with ABX treatment. (B) Changes of body weight during HFD feeding (black arrow indicates the beginning of HFD feeding; red arrow indicates 5 weeks after HFD feeding). (C) The weight of the whole body (BW), the liver, the eWAT, and the iBAT in HFD‐fed WT and bid −/− mice under ABX. (D) Representative images of hepatic H&E staining (×100) and Oil Red O staining (×200). (E,F) Triglyceride (TG) and total cholesterol (TC) levels in the liver (E) and the serum (F). (G) Hepatic messenger RNA (mRNA) level of fibroblast growth factor 21 (fgf21) and serum level of FGF21 in mice with designed genotype and diet. (H) Hepatic mRNA level of fgf21, and serum level of FGF21 in mice with designed genotype and diet in combination with ABX treatment. Data are shown as means ± SEM. Groups with different letters had significant differences (p < 0.05); one‐way analysis of variance (ANOVA) followed by Duncan's post‐hoc test.
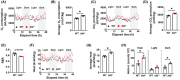
HFD‐fed bid −/− mice have a metabolic pattern favoring low body mass. Male WT and bid‐deficient (bid −/− ) mice (n = 3) were fed with HFD for 10 weeks and subjected to indirect calorimetry analysis for 48 h after initial acclimation. (A–D) Dynamic O2 consumption (A), average O2 consumption (B), dynamic CO2 generation (C), and average CO2 generation (D) were measured. (E) The respiratory exchange ratio (RER) was calculated by the ratio between the average of CO2 generation and the average consumption of O2. (F,H) Dynamic heat generation (F), average heat generation (G), and average physical activity (H) were recorded. Physical activity was represented by the ambulatory activity in three axes (Amb+Z) during a 48‐h period. Light time period was from 7:00 to 19:00; dark time period was from 19:00 to 7:00. Data are shown as means ± SEM. Groups with asterisks had significant differences (p < 0.05).
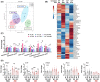
HFD‐fed bid −/− mice have altered hepatic metabolite profiles in lipid metabolism. Male WT and bid −/− mice were on RD or HFD for 10 weeks with or without ABX treatment (Figure 4A). (A) Livers from RD‐fed or HFD‐fed mice were subjected to metabolomics. Identified metabolites were subjected to sparse partial least‐squares discriminant analysis (sPLS‐DA). (B) Heat map shows metabolites with significant level changes among four groups (ANOVA; p < 0.05). sPLS‐DA plots and heat map were generated by MetaboAnalyst 5.0. Data are shown as average log2 bin. (C) The levels of certain fatty acids in WT or bid −/− livers following RD or HFD in mice given water or ABX treatment. (D) Hepatic expression of genes related to lipid synthesis was assessed with or without ABX treatment. Data are shown as means ± SEM. Statistical analysis was performed by one‐way ANOVA followed by Duncan's post‐hoc test. Groups with different letters had significant differences (p < 0.05). Acaca, acetyl‐CoA carboxylase alpha; Fasn, fatty acid synthase; Scd1, stearoyl‐CoA desaturase 1; Srebp1/2, sterol regulatory element‐binding transcription factor 1/2.
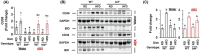
bid −/− mice were resistant to increase of hepatic CD36 induced by HFD. Male WT and bid −/− mice were on RD or HFD for 10 weeks with or without ABX treatment (Figure 4A). (A) Expression of cluster of differentiation 36 (Cd36) mRNA in the liver following HFD feeding. (B,C) Hepatic CD36 protein levels were analyzed by immunoblotting (B) and densitometry (C) in mice following HFD feeding in the absence or presence of ABX. Data are shown as means ± SEM. Statistical analysis was performed by one‐way ANOVA followed by Duncan's post‐hoc test. Groups with different letters had significant differences (p < 0.05).
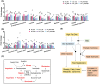
HFD‐fed bid −/− mice have altered hepatic profiles of intermediate metabolites. Male WT and bid −/− mice were on RD or HFD for 10 weeks with or without ABX treatment (Figure 4A). (A,B) The levels of certain sugar metabolites (A) or intermediate metabolites (B) in WT or bid −/− livers following RD or HFD, with or without ABX treatment. (C) A simplified diagram of trichloroacetic acid (TCA) cycle showing the conversion of the relevant intermediate metabolites. (D) A working model of the interplay among the various factors that can affect diet‐induced obesity and metabolic syndrome. Black dots indicate unknown mechanisms by which BID, altered microbial, and possibly other factors could affect the host under HFD. It is possible that BID could modify the host response to altered microbial, leading to changes in metabolism of lipids and other intermediate metabolites, which ultimately affect the obesity phenotype. The pathway regulated by sex hormones, but not that by increased food uptake (leptin deficiency), may likely converge with that of BID. Data are shown as means ± SEM. Statistical analysis was performed by one‐way ANOVA followed by Duncan's post‐hoc test. Groups with asterisks had significant differences (p < 0.05). CoA, coenzyme A.
Similar articles
-
Vezza T, Rodríguez-Nogales A, Algieri F, Garrido-Mesa J, Romero M, Sánchez M, Toral M, Martín-García B, Gómez-Caravaca AM, Arráez-Román D, Segura-Carretero A, Micol V, García F, Utrilla MP, Duarte J, Rodríguez-Cabezas ME, Gálvez J. Vezza T, et al. Pharmacol Res. 2019 Dec;150:104487. doi: 10.1016/j.phrs.2019.104487. Epub 2019 Oct 11. Pharmacol Res. 2019. PMID: 31610229
-
Porras D, Nistal E, Martínez-Flórez S, Pisonero-Vaquero S, Olcoz JL, Jover R, González-Gallego J, García-Mediavilla MV, Sánchez-Campos S. Porras D, et al. Free Radic Biol Med. 2017 Jan;102:188-202. doi: 10.1016/j.freeradbiomed.2016.11.037. Epub 2016 Nov 25. Free Radic Biol Med. 2017. PMID: 27890642
-
de Sant'Ana LP, Ribeiro DJS, Martins AMA, Dos Santos FN, Corrêa R, Almeida RDN, Eberlin MN, Maurice CF, Magalhães KG. de Sant'Ana LP, et al. Front Immunol. 2020 Jan 9;10:2926. doi: 10.3389/fimmu.2019.02926. eCollection 2019. Front Immunol. 2020. PMID: 31998283 Free PMC article.
-
Zheng Y, Wu Y, Tao L, Chen X, Jones TJ, Wang K, Hu F. Zheng Y, et al. Nutrients. 2020 Mar 30;12(4):959. doi: 10.3390/nu12040959. Nutrients. 2020. PMID: 32235581 Free PMC article.
-
Dietary fat and gut microbiota interactions determine diet-induced obesity in mice.
Kübeck R, Bonet-Ripoll C, Hoffmann C, Walker A, Müller VM, Schüppel VL, Lagkouvardos I, Scholz B, Engel KH, Daniel H, Schmitt-Kopplin P, Haller D, Clavel T, Klingenspor M. Kübeck R, et al. Mol Metab. 2016 Oct 13;5(12):1162-1174. doi: 10.1016/j.molmet.2016.10.001. eCollection 2016 Dec. Mol Metab. 2016. PMID: 27900259 Free PMC article.
References
-
- Jensen MD, Ryan DH, Apovian CM, Ard JD, Comuzzie AG, et al. 2013 AHA/ACC/TOS Guideline for the Management of Overweight and Obesity in Adults. J Am Coll Cardiol. 2014;63:2985–3023. - PubMed
-
- Finkelstein EA, Trogdon JG, Cohen JW, Dietz W. Annual medical spending attributable to obesity: payer‐and service‐specific estimates. Health Aff (Millwood). 2009;28:w822–31. - PubMed
-
- Adams LA, Anstee QM, Tilg H, Targher G. Non‐alcoholic fatty liver disease and its relationship with cardiovascular disease and other extrahepatic diseases. Gut. 2017;66:1138–53. - PubMed
-
- Cotter TG, Rinella M. Nonalcoholic fatty liver disease 2020: the state of the disease. Gastroenterology. 2020;158:1851–64. - PubMed
Publication types
MeSH terms
Substances
LinkOut - more resources
Full Text Sources
Medical