Physiology of the tongue with emphasis on taste transduction - PubMed
- ️Sun Jan 01 2023
Review
Physiology of the tongue with emphasis on taste transduction
Máire E Doyle et al. Physiol Rev. 2023.
Abstract
The tongue is a complex multifunctional organ that interacts and senses both interoceptively and exteroceptively. Although it is easily visible to almost all of us, it is relatively understudied and what is in the literature is often contradictory or is not comprehensively reported. The tongue is both a motor and a sensory organ: motor in that it is required for speech and mastication, and sensory in that it receives information to be relayed to the central nervous system pertaining to the safety and quality of the contents of the oral cavity. Additionally, the tongue and its taste apparatus form part of an innate immune surveillance system. For example, loss or alteration in taste perception can be an early indication of infection as became evident during the present global SARS-CoV-2 pandemic. Here, we particularly emphasize the latest updates in the mechanisms of taste perception, taste bud formation and adult taste bud renewal, and the presence and effects of hormones on taste perception, review the understudied lingual immune system with specific reference to SARS-CoV-2, discuss nascent work on tongue microbiome, as well as address the effect of systemic disease on tongue structure and function, especially in relation to taste.
Keywords: SARS-CoV-2; hormones; mechanisms of taste; stem cell; taste.
Conflict of interest statement
No conflicts of interest, financial or otherwise, are declared by the authors.
Figures
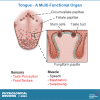
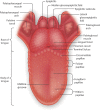
Gross anatomy of the human tongue emphasizing the locations of taste papillae. The tongue is divided transversely into the root or radix lingue (the posterior third) and the body of the tongue or corpus lingue (the anterior two-thirds). The root of the tongue is a papilla-free mucosa, covered with mucous glands and lymphatic tissue, referred to as the lingual tonsil. The 3 types of taste papillae are located in the body of the tongue. Circumvallate papillae are in a V-shaped arrangement at the back of the tongue. The foliate papillae consist of some ridges and slits generally arranged irregularly along the sides of the tongue. The fungiform papillae are the most numerous of the taste papillae and are located on the anterior surface of the tongue interspersed with filiform papillae. Both types of papillae are sparse along the lingual margin and abundant in the middle regions.
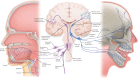
Taste sensation and sensory information are transmitted by cranial nerves to gustatory and sensory cortices though nuclei in the brainstem and thalamus. Gustatory information is relayed to the cemtral nervous system through gustatory nerve fibers from branches of 3 cranial nerves: chorda tympani branch of the facial (CN VII), glossopharyngeal (CN IX), and the superior laryngeal branch of the vagal nerve (CN X). Afferent gustatory nerve fibers converge in the gustatory area of the nucleus of the tractus solitarius, within the medulla of the brainstem. From there, second order neurons transmit information to the ventral posterior medial nucleus (VPM) of the thalamus and, from there, afferent neurons relay gustatory signals to the gustatory cortex. In the brainstem, above the pons, decussation of some second-order neurons occurs, and from there gustatory pathways ascend bilaterally. Touch-position and pain-temperature sensory information from the mouth is carried by branches of the trigeminal cranial nerve (CN V). Afferent neurons enter the brainstem and innervate the principal nucleus. From there, neurons project to VPM, and, finally, from the thalamus, sensory information is transmitted to the sensory cortex in the postcentral gyrus.
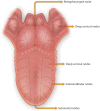
Lymphatic drainage of the dorsum and the base of the tongue. The mapped arrows show the direction of drainage of lymph fluid from the base of the tongue predominantly to the lymph nodes of levels II and III. Lymph fluid of the mucosa located around the midline flows vertically to the submandibular (anterior tongue) and upper jugular lymph nodes (posterior tongue) by way of 5 to 7 collectors located between the genioglossal muscles. Modified from Ref. , with permission from John Wiley & Sons.
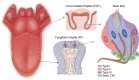
Taste is first discriminated in taste receptor cells (TRCs) within taste buds located in circumvallate papillae (CVP), foliate papillae (FLP), and fungiform papillae. By convention, 4 subtypes of taste bud cells are present in taste buds. Of the 4 subtypes, only type III TRCs form synapses with the afferent nerve fibers, represented by the footplates shown in brown.
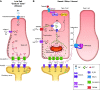
Signal transduction mechanism for sodium taste perception (A). The low-sodium-sensing cell is depolarized by the influx of Na+ via amiloride-sensitive epithelial sodium channel (ENaC). Additional influx of Na+ through voltage-gated sodium channel (VGNaC ) creates an action potential that ultimately leads to the release of ATP through CALHM1/3, without the involvement of intracellular calcium (Ca2+: 86,100). Mechanistic overview of signal transduction in sweet, bitter, and umami taste perception (B). Tastants bind to cell surface G protein-coupled receptors (GPCRs) and initiate a signaling cascade through phospholipase Cβ2 (PLCβ2) and inositol 1,4,5-triphosphate (IP3) that mobilizes Ca2+ from the endoplasmic reticulum (ER) by activating the IP3 receptor type 3 (IP3R3), thus increasing intracellular Ca2+ concentration. The spike in intracellular calcium activates transient receptor potential cation channel subfamily M member 5 (TRPM5) channels that depolarize the plasma membrane and create action potentials via voltage-gated Na+ channels (VGNa + C). These changes in the membrane potential and the increased presence of intracellular Ca2+ trigger the release of ATP into the channel synapse through the CALHM1/3s. This stimulates the gustatory neuron, thus completing transduction of the signal from the taste cell to the afferent (–88). Image created with BioRender.com, with permission.
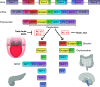
Tissue-specific proglucagon processing by different proconvertases yields different mature peptides in gut, brain, pancreas, and tongue. The proglucagon gene is composed of 6 exons and is transcribed and translated into a preproprotein containing a signal peptide (SP). Cleavage of the signal peptide results in the release of proglucagon, a 158 amino acid precursor protein that is found in the gut, brain, pancreas, and tongue. Posttranslational processing by different proconvertases results in multiple peptides. In enteroendocrine L cells of the ileum and colon, and in Glucagon-like peptide-1 (GLP-1)-producing (PPG) neurons in the nucleus of the tractus solitarius of the brainstem, where there is predominant expression of proconvertase 1/3 (PC 1/3), proglucagon is cleaved into glicentin, oxyntomodulin, GLP-1, GLP-2, and intervening peptide-2 (IP-2). In type II and type III taste receptor cells, and in α-cells in pancreatic islets, where both PC 1/3 and PC 2 are expressed, proglucagon is cleaved into glicentin-related pancreatic polypeptide (GRPP), glucagon, intervening peptide-1 (IP-1), major proglucagon fragment (MPGF), GLP-1, GLP-2, and IP-2. GLP-1 and glucagon are enhancers of sweet taste and are produced in type II cells (85, 111).
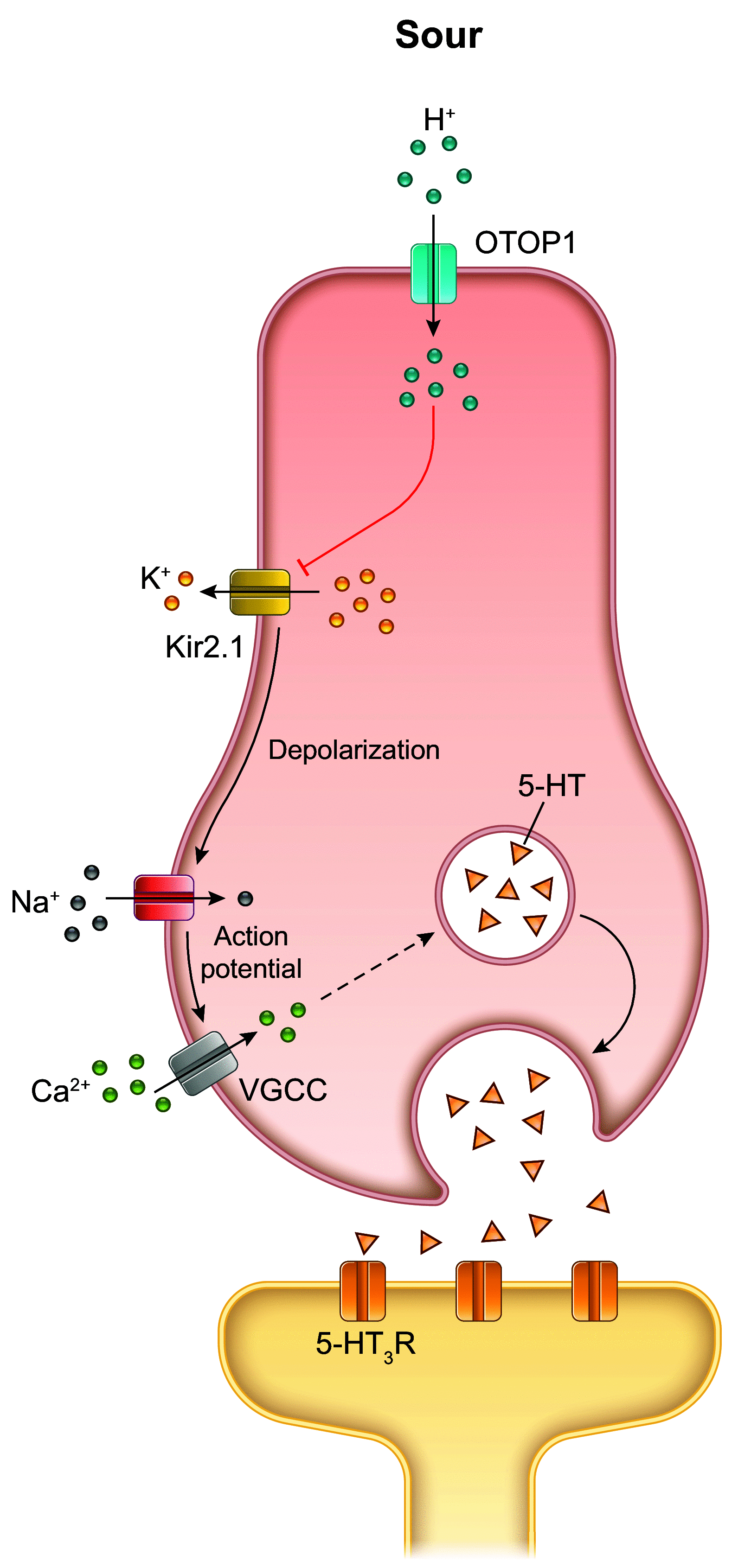
Mechanism of transduction of sour taste. OTOP1 is recognized as the sour receptor and conducts the H+ ions (protons) from acids into the cell cytosol. The influx of cations causes the membrane potential to change direction, and the change in intracellular pH blocks KIR2.1 K+ channels, which further depolarizes the membrane potential. With sufficient depolarization, voltage-gated Na+ channels open causing a train of action potentials that open voltage-gated calcium channels and lead to release of the neurotransmitter 5-HT. 5-HT released at the synapses activates the afferent nerve fibers via excitatory 5-HT3 receptors (R). Image created with BioRender.com, with permission.
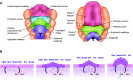
Mammalian tongue (A) and FP formation from the lingual epithelia layer (B). A: development of the mammalian tongue: fourth postovulatory week of human development is shown on the left and the 5-month stage is shown on the right-hand side. The anterior two-thirds of the tongue (shown in red) emerges from the lateral lingual swellings and tuberculum impar while the posterior third (shown in green) emerges from the copula. I–IV: position of the 5 pharyngeal arches. The posterior third of the tongue is derived from endoderm, whereas the anterior two-thirds of the tongue is derived from ectoderm, a fact that resonates through the many differences observed in innervation, taste bud composition, nature of taste receptor cells (TRC), and TRC renewal. B: schema showing early morphogenesis of fungiform papillae (FP) from taste placodes during mouse tongue development. 1: Morphogenesis of the taste placode begins at E12.5 through the epithelial-mesenchymal interaction signals [fibroblast growth factor 8 (Fgf8) and sonic hedgehog (shh) and its ligands Gli1 and Patched (Ptc) and bone morphogenic protein 2 and 4 (Bmp2 and 4)] from the epithelium are shown above in blue and those from the mesenchyme are shown below in red. 2: At E13, epithelial thickening occurs with the epithelial cells at the basal epithelium on the anterior part of the tongue increased in height. 3: At embryonic day (E) 13.5, the columnar epithelial cells begin to form an arch to which expression of the epithelial signaling molecules is restricted. 4: At E14, the FP commit to the next developmental phase with nerve innervations shown in green: neuronal innervation is required for taste bud development within the FP.
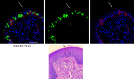
Human fungiform papillae (FP) showing taste bud and basal stem cell layer. Immunofluorescence stainings are of Ki67 (green, a marker of proliferating cells) and dipeptidyl peptidase (DDP; red). DPP4, also known as CD26 and adenine deaminase complexing protein 2, is a multifunctional membrane-bound and soluble protein that is best known for its activity as an exopeptidase. It is also a viral receptor (for MERS-CoV) and is involved in the regulation of intracellular signal transduction of T lymphocytes and apoptosis. DPP4 staining of individual cells, mostly likely T lymphocytes, can be seen in the lamina propria of the fungiform papillae and in all the stem cells of the basal layer, including the few cells directly underneath the taste bud. This is the first time that DPP4 has been shown to be present on membranes of this stem cell layer. No DPP4 is present in taste buds, and therefore mature taste receptor cells lose this cell surface marker. The arrow indicates the position of a taste bud (outlined in black), which can be seen in the hematoxylin and eosin staining image. FP biopsy was obtained from a young male in the fasted state. Scale bar = 50 µm.
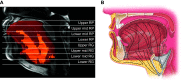
Tongue fat and lymphatic drainage. A: representative 3-dimensional volumetric reconstruction of tongue and fat within tongue (yellow) from a series of 3-mm contiguous axial MR images superimposed on a midsagittal image. There is substantially more fat at the base of the tongue. RG, retroglossal; RP, retropalatal. Image from Ref. , with permission from Oxford University Press. B: transverse section through the oral cavity illustrating lymphatic drainage of the tongue.
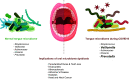
The tongue microbiome and its implications in human health. The healthy human tongue-coating microbiome is dominated by the genera Streptococcus, Veillonella, Actinomyces, Neisseria, and Prevotella; it is dysbiosis being associated with many other systemic diseases. The onset of COVID19 due to SARS-CoV2 infection and its prolonged symptoms are associated with enriched Veillonella and Prevotella species in the tongue-coating microbiome. Tongue microbiome dysbiosis is associated with local and systemic diseases. Image created with BioRender.com, with permission.
Similar articles
-
The sense of taste: Development, regeneration, and dysfunction.
Barlow LA. Barlow LA. WIREs Mech Dis. 2022 May;14(3):e1547. doi: 10.1002/wsbm.1547. Epub 2021 Nov 30. WIREs Mech Dis. 2022. PMID: 34850604 Free PMC article. Review.
-
What is the role of metabolic hormones in taste buds of the tongue.
Cai H, Maudsley S, Martin B. Cai H, et al. Front Horm Res. 2014;42:134-46. doi: 10.1159/000358322. Epub 2014 Apr 7. Front Horm Res. 2014. PMID: 24732931 Review.
-
[Research progress in functional regeneration methods and mechanisms of taste buds].
Wang JR, Li RQ, Huang XF. Wang JR, et al. Zhonghua Kou Qiang Yi Xue Za Zhi. 2024 Apr 9;59(4):389-393. doi: 10.3760/cma.j.cn112144-20231012-00190. Zhonghua Kou Qiang Yi Xue Za Zhi. 2024. PMID: 38548597 Chinese.
-
Mistretta CM, Kumari A. Mistretta CM, et al. Int J Mol Sci. 2019 Mar 16;20(6):1341. doi: 10.3390/ijms20061341. Int J Mol Sci. 2019. PMID: 30884865 Free PMC article. Review.
-
Danzer B, Jukic M, Dunkel A, Andersen G, Lieder B, Schaudy E, Stadlmayr S, Lietard J, Michel T, Krautwurst D, Haller B, Knolle P, Somoza M, Lingor P, Somoza V. Danzer B, et al. Sci Rep. 2024 Jul 4;14(1):15408. doi: 10.1038/s41598-024-66079-w. Sci Rep. 2024. PMID: 38965271 Free PMC article.
Cited by
-
Ankul SS, Chandran L, Anuragh S, Kaliappan I, Rushendran R, Vellapandian C. Ankul SS, et al. Front Pharmacol. 2023 Oct 24;14:1283440. doi: 10.3389/fphar.2023.1283440. eCollection 2023. Front Pharmacol. 2023. PMID: 37942488 Free PMC article.
-
Yata T, Aoyama N, Fujii T, Kida S, Taniguchi K, Iwane T, Tamaki K, Minabe M, Komaki M. Yata T, et al. J Clin Med. 2024 Aug 11;13(16):4711. doi: 10.3390/jcm13164711. J Clin Med. 2024. PMID: 39200853 Free PMC article.
-
Li YH, Yang YS, Xue YB, Lei H, Zhang SS, Qian J, Yao Y, Zhou R, Huang L. Li YH, et al. Elife. 2024 Jun 5;12:RP92956. doi: 10.7554/eLife.92956. Elife. 2024. PMID: 38836551 Free PMC article.
-
Lee S, Tochinai R, Yasuoka A, Nagai T, Saito Y, Kuwahara M, Abe K, Asakura T. Lee S, et al. Front Neurosci. 2023 Sep 14;17:1260655. doi: 10.3389/fnins.2023.1260655. eCollection 2023. Front Neurosci. 2023. PMID: 37781249 Free PMC article.
-
Lorena MDSV, Santos EKD, Ferretti R, Nagana Gowda GA, Odom GL, Chamberlain JS, Matsumura CY. Lorena MDSV, et al. Skelet Muscle. 2023 Sep 13;13(1):16. doi: 10.1186/s13395-023-00325-z. Skelet Muscle. 2023. PMID: 37705069 Free PMC article.
References
-
- Prescott J. Flavour as a psychological construct: implications for perceiving and measuring the sensory qualities of foods. Food Quality Pref 10: 349–356, 1999. doi:10.1016/S0950-3293(98)00048-2. - DOI
-
- Delwiche J. The impact of perceptual interactions on perceived flavor. Food Quality Prefer 15: 137–146, 2004. doi:10.1016/S0950-3293(03)00041-7. - DOI
Publication types
MeSH terms
LinkOut - more resources
Full Text Sources
Medical
Miscellaneous