SV40 T-antigen uses a DNA shearing mechanism to initiate origin unwinding - PubMed
- ️Sat Jan 01 2022
SV40 T-antigen uses a DNA shearing mechanism to initiate origin unwinding
Lance D Langston et al. Proc Natl Acad Sci U S A. 2022.
Abstract
Duplication of DNA genomes requires unwinding of the double-strand (ds) DNA so that each single strand (ss) can be copied by a DNA polymerase. The genomes of eukaryotic cells are unwound by two ring-shaped hexameric helicases that initially encircle dsDNA but transition to ssDNA for function as replicative helicases. How the duplex is initially unwound, and the role of the two helicases in this process, is poorly understood. We recently described an initiation mechanism for eukaryotes in which the two helicases are directed inward toward one another and shear the duplex open by pulling on opposite strands of the duplex while encircling dsDNA [L. D. Langston, M. E. O'Donnell, eLife 8, e46515 (2019)]. Two head-to-head T-Antigen helicases are long known to be loaded at the SV40 origin. We show here that T-Antigen tracks head (N-tier) first on ssDNA, opposite the direction proposed for decades. We also find that SV40 T-Antigen tracks directionally while encircling dsDNA and mainly tracks on one strand of the duplex in the same orientation as during ssDNA translocation. Further, two inward directed T-Antigen helicases on dsDNA are able to melt a 150-bp duplex. These findings explain the "rabbit ear" DNA loops observed at the SV40 origin by electron microscopy and reconfigure how the DNA loops emerge from the double hexamer relative to earlier models. Thus, the mechanism of DNA shearing by two opposing helicases is conserved in a eukaryotic viral helicase and may be widely used to initiate origin unwinding of dsDNA genomes.
Keywords: DNA shearing mechanism; SV40 T-Antigen helicase; origin activation; origin unwinding; replication origins.
Conflict of interest statement
The authors declare no competing interest.
Figures
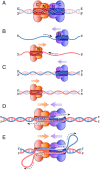
Essential features of the DNA shearing model of origin unwinding. (A) Two hexameric helicases are loaded head-to-head (N-face to N-face) surrounding dsDNA at origins of replication. The cutaway view shows the origin dsDNA inside the central channels of the two helicases. (B) The helicases are known to translocate 3′-5′ on ssDNA, and we show in this report that T-Ag is oriented N-first for translocation on ssDNA, as we found earlier for the CMG eukaryotic helicase (18). The colored arrows show the movement of the helicase with respect to the DNA, and the dotted black arrows show the movement of the DNA with respect to the helicase. (C) Even though the hexameric helicase encircles dsDNA, it makes primary contact with the same 3′–5′ tracking strand as in (B) for ssDNA. (D) Because the helicases are both surrounding dsDNA, they block each other’s N-first forward movement along the DNA, and we propose that the C-terminal AAA+ motors of each helicase (shown as hands pulling on the ssDNA) continue to track on their respective strands, shearing the duplex DNA apart between the two motors. (E) Continued tracking on their respective DNA strands leads to continued DNA shearing, with the unwound ssDNA spooling behind each helicase as growing ssDNA loops, while the distal ends of the duplex DNA are drawn toward the center. Image credit: Nina Yao.
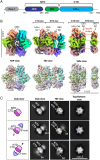
The N-tier of T-Ag faces toward the 5′ end of ssDNA. T-Ag containing amino acid residues 131–627 was incubated with AMP-PNP, either in the absence of DNA or in the presence of two separate 20-mer oligonucleotides, one of which is biotinylated at the 5′ end and the other at the 3′ end, as indicated by illustrations in panel C of the figure. Streptavidin (SA) was added after adding the T-Ag131–627. (A) Domain architecture of T-Ag. (B) Cryo-EM 3D map of T-Ag hexamer (Upper panel) and rigid-body docking with partial atomic models (Lower row; PDB ID 1SVM for AAA+ and ZBD, and 2FUF for OBD) shown in three orthogonal views. Subunits are individually colored. The OBD and ZBD domains, comprising the N-tier of T-Ag are connected to the C-tier region by a 14 residue disordered region, as indicated by squiggly lines between the OBD and the ZBD-AAA+ domains. (C) 2D class averages are shown for the side view, the tilted view, and the top view for each set of reactions. Comparison of the samples with biotin-SA-DNA to those without DNA indicates the presence of additional density representing SA at either the N- or C-tier of T-Ag depending on whether the oligo was biotinylated at the 3′ or 5′ end. The question mark in one panel indicates a density too light to definitively assign to SA.
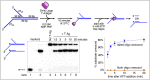
SV40 T-Antigen tracks directionally while encircling duplex DNA. The design of the substrate is shown in the schematic at Top, Left. The radiolabeled oligo in the substrate is indicated by an asterisk at the 5′ end. Wild-type T-Ag (40 nM as hexamer) is preincubated with the substrate (0.5 nM) for 10 min in the presence of 0.2 mM AMP-PNP before starting the reaction by addition of 5 mM ATP along with trap oligos to prevent reannealing of either the flush- or tailed-duplex oligo after unwinding (see Materials and Methods and
SI Appendixfor details). Lanes 1 and 2 in the native PAGE gel (Bottom Left) show the migration of the two potential products of unwinding. Lane 3 shows the unreacted substrate and lanes 4–8 show the time course of unwinding by T-Ag. The plot at the bottom right shows the time course of unwinding of the tailed duplex (blue circles) and flush duplex (orange circles). Values in the plot are the average of three independent experiments, and the error bars show the SD.
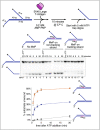
T-Ag mainly contacts one strand of dsDNA while moving directionally to unwind a downstream 5’ tailed DNA strand. The design of the substrate is shown in the reaction scheme at Top, Left. The radiolabeled oligo in the substrate is indicated by an asterisk at the 5’ end. As shown in pink on the schematics above the gel, the substrate contains 20 charge-neutralizing MeP linkages on the phosphate backbone of the tracking strand, (lanes 13–18), the nontracking strand (lanes 7–12) or neither strand (lanes 1–6). T-Ag (5 nM as hexamer) is preincubated with the substrate (0.5 nM) for 10 min in the presence of 0.2 nM AMP-PNP before starting the reaction by the addition of 5 mM ATP along with unlabeled trap DNA. The plot at the bottom shows the time course of unwinding of the substrate with no MePs (blue circles) or 20 MeP linkages on the tracking (gray circles) or nontracking (orange circles) strand. Values in the plot are the average of three independent experiments, and the error bars show the SD.
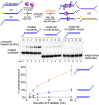
Two oppositely facing T-Ag hexamers can unwind duplex DNA. Loading of two T-Ag hexamers in opposing orientations leads to unwinding of nonorigin duplex DNA. A 150-bp duplex DNA with no SV40 origin sequences was assembled containing 3′ tails at one end or the other or at both ends. The radiolabeled oligo in the substrate is indicated by an asterisk at the 5’ end. T-Ag (40 nM as hexamer) is preincubated with the substrate (0.5 nM) for 10 min in the presence of 0.2 nM AMP-PNP before starting the reaction by the addition of 5 mM ATP along with trap oligos (shown in orange in the schematic at Top) that anneal to the unwound radiolabeled strand and shift it to a slower migrating position in the gel (see
SI Appendixfor details). The plot at the bottom shows the time course of unwinding of the substrate with two 3′ tails (orange circles) or a single 3′ tail on the top or bottom strand (blue and gray circles, respectively). Values in the plot are the average of three independent experiments, and the error bars show the SD.
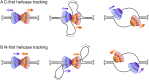
Contrast between C-first and N-first T-Ag helicase tracking at origins. The first illustrations of both panels (A and B) show the head-to-head (N-to-N) double hexamer of T-Ag, but the arrows denote whether they: (A) move C-first in opposite directions on DNA, or (B) move N-first into one another in which case they must pass each another on ssDNA. For two T-Ag hexamers that track C-first and simply depart in opposite directions, the observation of “rabbit ear” DNA loops suggests that the two T-Ag must remain connected together and draw DNA toward them to form the ssDNA loops. However, N-first tracking does not require long-lasting direct connections among T-Ag hexamers, and it pits the two T-Ag against one another to shear DNA and form “rabbit ear” ssDNA loops. In the case of N-first tracking of T-Ag, the loops would be expelled out the back of the C-terminal motor domains. N-first T-Ag hexamers track on ssDNA upon expulsion of the nontracking strand. Image credit: Nina Yao (Rockefeller University, New York, NY).
Similar articles
-
Wan L, Toland S, Robinson-McCarthy LR, Lee N, Schaich MA, Hengel SR, Li X, Bernstein KA, Van Houten B, Chang Y, Moore PS. Wan L, et al. Proc Natl Acad Sci U S A. 2023 Jul 25;120(30):e2308010120. doi: 10.1073/pnas.2308010120. Epub 2023 Jul 17. Proc Natl Acad Sci U S A. 2023. PMID: 37459531 Free PMC article.
-
Mechanism of eukaryotic origin unwinding is a dual helicase DNA shearing process.
Langston LD, Georgescu RE, O'Donnell ME. Langston LD, et al. Proc Natl Acad Sci U S A. 2023 Dec 26;120(52):e2316466120. doi: 10.1073/pnas.2316466120. Epub 2023 Dec 18. Proc Natl Acad Sci U S A. 2023. PMID: 38109526 Free PMC article.
-
An explanation for origin unwinding in eukaryotes.
Langston LD, O'Donnell ME. Langston LD, et al. Elife. 2019 Jul 8;8:e46515. doi: 10.7554/eLife.46515. Elife. 2019. PMID: 31282859 Free PMC article.
-
Patel SS, Pandey M, Nandakumar D. Patel SS, et al. Curr Opin Chem Biol. 2011 Oct;15(5):595-605. doi: 10.1016/j.cbpa.2011.08.003. Epub 2011 Aug 22. Curr Opin Chem Biol. 2011. PMID: 21865075 Free PMC article. Review.
-
Contacts and context that regulate DNA helicase unwinding and replisome progression.
Perera HM, Behrmann MS, Hoang JM, Griffin WC, Trakselis MA. Perera HM, et al. Enzymes. 2019;45:183-223. doi: 10.1016/bs.enz.2019.08.001. Epub 2019 Sep 12. Enzymes. 2019. PMID: 31627877 Review.
Cited by
-
The Response of the Replication Apparatus to Leading Template Strand Blocks.
Bellani MA, Shaik A, Majumdar I, Ling C, Seidman MM. Bellani MA, et al. Cells. 2023 Nov 11;12(22):2607. doi: 10.3390/cells12222607. Cells. 2023. PMID: 37998342 Free PMC article. Review.
-
Wan L, Toland S, Robinson-McCarthy LR, Lee N, Schaich MA, Hengel SR, Li X, Bernstein KA, Van Houten B, Chang Y, Moore PS. Wan L, et al. Proc Natl Acad Sci U S A. 2023 Jul 25;120(30):e2308010120. doi: 10.1073/pnas.2308010120. Epub 2023 Jul 17. Proc Natl Acad Sci U S A. 2023. PMID: 37459531 Free PMC article.
-
Shin J, Meinke G, Bohm AA, Bullock PA. Shin J, et al. J Virol. 2024 Oct 22;98(10):e0111924. doi: 10.1128/jvi.01119-24. Epub 2024 Sep 23. J Virol. 2024. PMID: 39311578
-
Essential and multifunctional mpox virus E5 helicase-primase in double and single hexamer.
Xu Y, Wu Y, Zhang Y, Gao K, Wu X, Yang Y, Li D, Yang B, Zhang Z, Dong C. Xu Y, et al. Sci Adv. 2024 Aug 23;10(34):eadl1150. doi: 10.1126/sciadv.adl1150. Epub 2024 Aug 21. Sci Adv. 2024. PMID: 39167653 Free PMC article.
-
Unwinding of a eukaryotic origin of replication visualized by cryo-EM.
Henrikus SS, Gross MH, Willhoft O, Pühringer T, Lewis JS, McClure AW, Greiwe JF, Palm G, Nans A, Diffley JFX, Costa A. Henrikus SS, et al. Nat Struct Mol Biol. 2024 Aug;31(8):1265-1276. doi: 10.1038/s41594-024-01280-z. Epub 2024 May 17. Nat Struct Mol Biol. 2024. PMID: 38760633 Free PMC article.
References
Publication types
MeSH terms
Substances
LinkOut - more resources
Full Text Sources