Contribution of Glial Cells to Polyglutamine Diseases: Observations from Patients and Mouse Models - PubMed
Review
Contribution of Glial Cells to Polyglutamine Diseases: Observations from Patients and Mouse Models
Marija Cvetanovic et al. Neurotherapeutics. 2023 Jan.
Abstract
Neurodegenerative diseases are broadly characterized neuropathologically by the degeneration of vulnerable neuronal cell types in a specific brain region. The degeneration of specific cell types has informed on the various phenotypes/clinical presentations in someone suffering from these diseases. Prominent neurodegeneration of specific neurons is seen in polyglutamine expansion diseases including Huntington's disease (HD) and spinocerebellar ataxias (SCA). The clinical manifestations observed in these diseases could be as varied as the abnormalities in motor function observed in those who have Huntington's disease (HD) as demonstrated by a chorea with substantial degeneration of striatal medium spiny neurons (MSNs) or those with various forms of spinocerebellar ataxia (SCA) with an ataxic motor presentation primarily due to degeneration of cerebellar Purkinje cells. Due to the very significant nature of the degeneration of MSNs in HD and Purkinje cells in SCAs, much of the research has centered around understanding the cell autonomous mechanisms dysregulated in these neuronal cell types. However, an increasing number of studies have revealed that dysfunction in non-neuronal glial cell types contributes to the pathogenesis of these diseases. Here we explore these non-neuronal glial cell types with a focus on how each may contribute to the pathogenesis of HD and SCA and the tools used to evaluate glial cells in the context of these diseases. Understanding the regulation of supportive and harmful phenotypes of glia in disease could lead to development of novel glia-focused neurotherapeutics.
Keywords: Glia; Huntington’s disease; Polyglutamine; Spinocerebellar ataxia.
© 2023. The American Society for Experimental Neurotherapeutics, Inc.
Figures
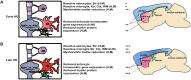
Huntington’s disease and glial cells. A Left—early-stage HD (H) and mutant Huntingtin (yellow) expressing mouse (M) brains. Reactive astrocytes (red) and reactive microglia (green) are present at early stages of HD, and myelin integrity (oligodendrocytes [purple]) is reduced. Gene expression changes are present at early disease stages. Top right—Reduced astrocytic homeostatic gene expression (red and blue) and myelin protein expression (green) is at early HD stages in cortex, striatum, and white matter. B Left—The number of reactive astrocytes (red) and reactive microglia (green) increases more at later HD stages, and myelin integrity (oligodendrocytes [purple]) is further reduced. Right—Astrocyte gene expression changes (red and blue) and myelin protein reduction (green) are exacerbated at later disease stages
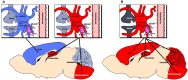
A Early stages of SCA1. Brain regions indicated in blue exhibit increased (cortex) or trending (cerebellum) expression of astrocyte (blue cells) homeostatic genes likely providing increased support to neurons (gray), whereas regions in red exhibit loss of astrocyte (red astrocytes) homeostatic function (hippocampus and medulla oblongata). B Late, terminal stages of SCA1. Astrocytes in all brain regions exhibit reduced expression of genes necessary for astrocyte homeostatic function (cortex, cerebellum, hippocampus, and medulla). Microglia activation (pink) in different brain regions does not correlate with astrocyte reactivity or homeostatic gene expression. Yellow triangles represent expression of Atxn1 gene in all these cell types in mice and ATXN1 humans
Similar articles
-
Onur TS, Laitman A, Zhao H, Keyho R, Kim H, Wang J, Mair M, Wang H, Li L, Perez A, de Haro M, Wan YW, Allen G, Lu B, Al-Ramahi I, Liu Z, Botas J. Onur TS, et al. Elife. 2021 Apr 19;10:e64564. doi: 10.7554/eLife.64564. Elife. 2021. PMID: 33871358 Free PMC article.
-
Buckner N, Kemp KC, Scott HL, Shi G, Rivers C, Gialeli A, Wong LF, Cordero-LLana O, Allen N, Wilkins A, Uney JB. Buckner N, et al. Brain Pathol. 2020 Nov;30(6):1041-1055. doi: 10.1111/bpa.12872. Epub 2020 Jul 13. Brain Pathol. 2020. PMID: 32580238 Free PMC article.
-
Bezprozvanny I. Bezprozvanny I. Neurochem Res. 2011 Jul;36(7):1186-97. doi: 10.1007/s11064-010-0393-y. Epub 2011 Jan 6. Neurochem Res. 2011. PMID: 21210219 Free PMC article. Review.
-
Yang Y, Yang S, Guo J, Cui Y, Tang B, Li XJ, Li S. Yang Y, et al. J Neurosci. 2017 Sep 20;37(38):9101-9115. doi: 10.1523/JNEUROSCI.0111-17.2017. Epub 2017 Aug 18. J Neurosci. 2017. PMID: 28821675 Free PMC article.
-
The contribution of glial cells to Huntington's disease pathogenesis.
Wilton DK, Stevens B. Wilton DK, et al. Neurobiol Dis. 2020 Sep;143:104963. doi: 10.1016/j.nbd.2020.104963. Epub 2020 Jun 25. Neurobiol Dis. 2020. PMID: 32593752 Free PMC article. Review.
Cited by
-
A New Look at Animal Models of Neurological Disorders.
Chesselet MF. Chesselet MF. Neurotherapeutics. 2023 Jan;20(1):1-2. doi: 10.1007/s13311-023-01366-4. Epub 2023 Apr 5. Neurotherapeutics. 2023. PMID: 37017897 Free PMC article. No abstract available.
References
Publication types
MeSH terms
Substances
LinkOut - more resources
Full Text Sources
Medical