Role of the kidneys in acid-base regulation and ammonia excretion in freshwater and seawater fish: implications for nephrocalcinosis - PubMed
- ️Sun Jan 01 2023
Review
Role of the kidneys in acid-base regulation and ammonia excretion in freshwater and seawater fish: implications for nephrocalcinosis
Marius Takvam et al. Front Physiol. 2023.
Abstract
Maintaining normal pH levels in the body fluids is essential for homeostasis and represents one of the most tightly regulated physiological processes among vertebrates. Fish are generally ammoniotelic and inhabit diverse aquatic environments that present many respiratory, acidifying, alkalinizing, ionic and osmotic stressors to which they are able to adapt. They have evolved flexible strategies for the regulation of acid-base equivalents (H+, NH4 +, OH- and HCO3 -), ammonia and phosphate to cope with these stressors. The gills are the main regulatory organ, while the kidneys play an important, often overlooked accessory role in acid-base regulation. Here we outline the kidneys role in regulation of acid-base equivalents and two of the key 'urinary buffers', ammonia and phosphate, by integrating known aspects of renal physiology with recent advances in the molecular and cellular physiology of membrane transport systems in the teleost kidneys. The renal transporters (NHE3, NBC1, AE1, SLC26A6) and enzymes (V-type H+ATPase, CAc, CA IV, ammoniagenic enzymes) involved in H+ secretion, bicarbonate reabsorption, and the net excretion of acidic and basic equivalents, ammonia, and inorganic phosphate are addressed. The role of sodium-phosphate cotransporter (Slc34a2b) and rhesus (Rh) glycoproteins (ammonia channels) in conjunction with apical V-type H+ ATPase and NHE3 exchangers in these processes are also explored. Nephrocalcinosis is an inflammation-like disorder due to the precipitation of calcareous material in the kidneys, and is listed as one of the most prevalent pathologies in land-based production of salmonids in recirculating aquaculture systems. The causative links underlying the pathogenesis and etiology of nephrocalcinosis in teleosts is speculative at best, but acid-base perturbation is probably a central pathophysiological cause. Relevant risk factors associated with nephrocalcinosis are hypercapnia and hyperoxia in the culture water. These raise internal CO2 levels in the fish, triggering complex branchial and renal acid-base compensations which may promote formation of kidney stones. However, increased salt loads through the rearing water and the feed may increase the prevalence of nephrocalcinosis. An increased understanding of the kidneys role in acid-base and ion regulation and how this relates to renal diseases such as nephrocalcinosis will have applied relevance for the biologist and aquaculturist alike.
Keywords: acid-base regulation; aquaculture; fish; kidney stones; nephron tubule; patophysiology; transporters and channels; water quality (WQ).
Copyright © 2023 Takvam, Wood, Kryvi and Nilsen.
Conflict of interest statement
The authors declare that the research was conducted in the absence of any commercial or financial relationships that could be construed as a potential conflict of interest.
Figures
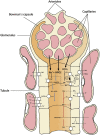
Schematic overview showing the main transport directions of acidic (H+, NH4 +) and basic (HCO3 -) equivalents and the uncharged respiratory gases CO2 (acidic) and NH3 (alkaline) involved in acid-base homeostasis in the teleost kidney. The primary urine filtrate from the glomerulus (and bowman`s capsule) contains bicarbonate (HCO3 -), sodium (Na+) and dibasic phosphate (HPO4 2-) that enters the tubule for either excretion or reabsorption as they move along the proximal and distal tubule and collecting duct. Extracellular carbonic anhydrase (CA-IV) on the tubule apical membrane facing the lumen catalyzes the dehydration reaction of a filtered HCO3 - (appearing in the primary urine) with a H+ secreted by the tubule cell. The H+ originates from the action of intracellular cytosolic carbonic anhydrase (CAc) that catalyzes the hydration of CO2, thereby permitting the HCO3 - ion to be returned to the blood. The back diffusion of CO2 from the urine to the tubule refuels this reaction. The uncharged gases (CO2 and NH3) move easily across cell membranes, whereas the movements of the charged ions HCO3 - and NH4 + must be facilitated by transporters or channels. The two major “urinary buffers” are ammonia and phosphate. Once most of the HCO3 - has been reabsorbed, any additional H+ ions that are secreted stay in the urine, titrating NH3 to NH4 +, and dibasic phosphate (HPO4 2-) to monobasic phosphate (H2PO4 -), both of which are excreted. Another mechanism of NH4 + appearance in the urine is also shown and may be quantitatively more important, based on mammalian studies. This is the oxidative metabolism of glutamine (or other amino acids) which produces equimolar amounts of NH4 + which is secreted into the urine, and HCO3 - which is returned to the blood plasma. Note that by both of these schemes, for every acidic equivalent excreted, a basic equivalent (HCO3 -) is added to the blood plasma. The different transport mechanisms occur in several segments of the nephron and the model is not intended to illustrate any specific segment, only the mechanisms.
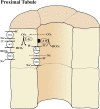
Basic transport mechanisms involved in reabsorption of bicarbonate (HCO3 -) in the proximal tubule of teleost kidney. The basolateral Na+- K+ -ATPase (NKA) and apical V-type H+-ATPase generate favorable transepithelial membrane potentials used by some of the other ion transport pathways. Extracellular carbonic anhydrase (CA-IV) on the tubule membrane dehydrates HCO3 - in the glomerular filtrate (primary urine) utilizing the H+ secreted by an apical Na+/H+ exchanger (NHE3) and/or by a V-type H+-ATPase coupled to a Na+ channel. The NHE3 is an abundant but less powerful secondary transport mechanism that cannot secrete H+ against low urine pH. The H+-ATPase is a less abundant but powerful enzymatic transport mechanism that can secrete against very low urine pH. Inside tubule cells cytosolic (CAc) catalyzes the hydration of CO2 forming HCO3 - to be returned to the blood via a basolateral Na+-HCO3 - co-transporter (NBC1) and/or anion exchanger AE1. If the Na+-coupled secretion mechanisms (NHE3, H+-ATPase) for H+ secretion cannot match the rate of HCO3 - filtration, then net HCO3 - excretion occurs, while if the H+ secretion rate exceeds the rate of HCO3 - filtration, then net H+ excretion occurs. Note that after all HCO3 - is reabsorbed continued net H+ excretion is dependent on the presence of urinary buffers (see figure 1).
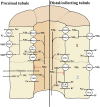
Basic transport mechanisms involved in secretion of ammonia in the proximal and distal/collecting tubule of teleost kidney. The basolateral Na+- K+ -ATPase (NKA) and apical V-type H+-ATPase generate favorable transepithelial membrane potentials used by some of the other ion transport pathways in the proximal and distal/collecting tubule (left side and right side; 1 and 2). The rhesus (Rh) glycoproteins are channels that facilitate the diffusion of ammonia; although NH3 is shown as the form moving through them, there is considerable uncertainty as to whether they facilitate the movement of NH3, NH4 +, or both. Rhbg and Rhcg1a enable the basolateral uptake of ammonia. Secretion of NH3 into the proximal tubule lumen is facilitated by apically located Rhcg1b and/or Rhcg1a channels (left side). Apical V-type H+ ATPase (proton pump) and NHE3 (Na+/H+ exchanger) both may transport H+ to the lumen, enabling the “diffusion trapping” mechanism (NH3 + H+) to form NH4 +. The diffusion trapping mechanism is important to avoid NH3 reabsorption back to the tubule cells and maintains the gradient for NH3 secretion, ensuring that NH4 + can ultimately be excreted through the urine. In the proximal tubule, NHE3 may play a dominant role, not only exchanging H+ against Na+ for diffusion trapping but also directly exchanging NH4 + (from the oxidation of glutamine and other amino acids) for Na+ (left side). The NHE3-mediated diffusion trapping mechanism is also found in the distal/collecting tubule; there, the Rhcg1a channels perform the basolateral uptake of ammonia (right side; 1). However, in the distal/collecting tubule a second mechanism is present (right side; 2). Here the basolateral uptake of ammonia is possible through the Rhbg channel and apical secretion via the apical Rhcg1b. The diffusion trapping mechanism is possible via the H+, K+ ATPase, providing the apical H+ secretion needed to form NH4 + for later excretion in the urine. Both NH4 + substitutions on the K+ site of the basolateral Na+, K+ ATPase and a HCN2 (a hyperpolarization-activated cyclic nucleotide gated channel (subtype 2)) have also been hypothesized as a possible NH4 + basolateral uptake mechanisms in the collecting duct (not shown).
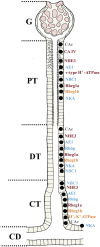
Overview of the localization of the transporters involved in acid-base, ammonia and phosphate regulation in teleost fish. Based on the current understanding and molecular analyses in teleosts, putative locations of various transporters in the nephron are presented for fish (G; Glomerulus, PT; Proximal tubule, DT; Distal tubule, CT; Collecting tubule and CD; Collecting duct). For the regulation pattern in different situations (e.g. changes in acid-base status, water chemistry, fasted versus non-fasted states) the reader is referred to the text. The CAc are found in all sections of the nephron PT, DT and CT. While similar location has been found for the CA IV it is not present in the CT. The NHE3, NBC1 AE1, Rhcg (1a and 1b), Rhbg and NKA are found in all segments. The V-type H+ ATPase are found only in the PT, and the H+, K+ ATPase only in the CT. Apical (orange), basolateral (blue) and multiple locations (dark red) are shown. The CAc (grey) is found in the cytosol of the cell.
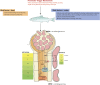
Hypothesis on the physiological and molecular mechanisms leading to the formation and aggregation of kidney stones in nephrocalcinosis. During elevated PCO2 and PO2 levels fish will experience chronic respiratory acidosis where blood pH is reduced and where the pH has been compensated by HCO3 - retention for which the kidney tubules have a key compensatory role. If water PCO2 and PO2 drop in the tank then PCO2 in the blood plasma and primary urine will quickly fall while HCO3 - in the plasma and primary urine will stay high for some time. The pH will quickly rise in the blood plasma and primary urine during this period, creating optimal conditions for nucleation, precipitation, and aggregation events to occur in urine that still has elevated Ca2+, Mg2+, NH4 +, HPO4 2- and HCO3 - concentrations. During this event the kidney stones will start forming at pH above 6.8 (carbonate apatite; Ca10(PO4)6CO3) and 7.2 (struvite; MgNH4PO4 ·6H2O). This may be the proximate trigger mechanism. Increased salt load in the water (12-15 ppt), salted feed and/or calcium and sodium bicarbonate buffers may further increase the Ca2+, Mg2+ and HCO3 - having a cumulative effect. For more details regarding the transport pathways the reader is referred to Figure 1, Figure 2, Figure 3.
Similar articles
-
Fehsenfeld S, Wood CM. Fehsenfeld S, et al. Am J Physiol Renal Physiol. 2018 Dec 1;315(6):F1565-F1582. doi: 10.1152/ajprenal.00510.2017. Epub 2018 Aug 8. Am J Physiol Renal Physiol. 2018. PMID: 30089033
-
Wright PA, Wood CM, Wilson JM. Wright PA, et al. J Exp Biol. 2014 Aug 15;217(Pt 16):2855-65. doi: 10.1242/jeb.098640. Epub 2014 May 22. J Exp Biol. 2014. PMID: 24855681
-
Lawrence MJ, Wright PA, Wood CM. Lawrence MJ, et al. J Exp Biol. 2015 Jul;218(Pt 13):2124-35. doi: 10.1242/jeb.117689. Epub 2015 May 18. J Exp Biol. 2015. PMID: 25987732
-
A new paradigm for ammonia excretion in aquatic animals: role of Rhesus (Rh) glycoproteins.
Wright PA, Wood CM. Wright PA, et al. J Exp Biol. 2009 Aug;212(Pt 15):2303-12. doi: 10.1242/jeb.023085. J Exp Biol. 2009. PMID: 19617422 Review.
-
An Update on Kidney Ammonium Transport Along the Nephron.
Harris AN, Skankar M, Melanmed M, Batlle D. Harris AN, et al. Adv Kidney Dis Health. 2023 Mar;30(2):189-196. doi: 10.1053/j.akdh.2022.12.005. Adv Kidney Dis Health. 2023. PMID: 36868733 Review.
Cited by
-
Mechanisms and physiological relevance of acid-base exchange in functional units of the kidney.
Gantsova E, Serova O, Vishnyakova P, Deyev I, Elchaninov A, Fatkhudinov T. Gantsova E, et al. PeerJ. 2024 Apr 29;12:e17316. doi: 10.7717/peerj.17316. eCollection 2024. PeerJ. 2024. PMID: 38699185 Free PMC article. Review.
References
-
- Arciuli M., Brunetti A., Fiocco D., Zacchino V., Centoducati G., Aloi A., et al. (2015). A multidisciplinary study of the extracutaneous pigment system of European sea bass (Dicentrarchus labrax L). A possible relationship between kidney disease and dopa oxidase activity level. Fish Shellfish Immunol. 42 (1), 184–192. 10.1016/j.fsi.2014.10.031 - DOI - PubMed
-
- Beamish F. W. H., Tandler A. (1990). Ambient ammonia, diet and growth in lake trout. Aquat. Toxicol. 17 (2), 155–166. 10.1016/0166-445X(90)90028-N - DOI
-
- Becke C., Schumann M., Geist J., Brinker A. (2020). Shape characteristics of suspended solids and implications in different salmonid aquaculture production systems. Aquaculture 516, 734631. 10.1016/j.aquaculture.2019.734631 - DOI
Publication types
Grants and funding
CW’s contribution was supported by NSERC (Canada) Discovery grants (RGPIN-2017-03843 and RGPIN-2023-03714). TN, MT, and HK’s contribution was supported by Norwegian Research council (NORDFORSK-332568).
LinkOut - more resources
Full Text Sources
Miscellaneous