The Influenza B Virus Victoria and Yamagata Lineages Display Distinct Cell Tropism and Infection-Induced Host Gene Expression in Human Nasal Epithelial Cell Cultures - PubMed
- ️Sun Jan 01 2023
The Influenza B Virus Victoria and Yamagata Lineages Display Distinct Cell Tropism and Infection-Induced Host Gene Expression in Human Nasal Epithelial Cell Cultures
Jo L Wilson et al. Viruses. 2023.
Abstract
Understanding Influenza B virus infections is of critical importance in our efforts to control severe influenza and influenza-related diseases. Until 2020, two genetic lineages of influenza B virus-Yamagata and Victoria-circulated in the population. These lineages are antigenically distinct, but the differences in virus replication or the induction of host cell responses after infection have not been carefully studied. Recent IBV clinical isolates of both lineages were obtained from influenza surveillance efforts of the Johns Hopkins Center of Excellence in Influenza Research and Response and characterized in vitro. B/Victoria and B/Yamagata clinical isolates were recognized less efficiently by serum from influenza-vaccinated individuals in comparison to the vaccine strains. B/Victoria lineages formed smaller plaques on MDCK cells compared to B/Yamagata, but infectious virus production in primary human nasal epithelial cell (hNEC) cultures showed no differences. While ciliated epithelial cells were the dominant cell type infected by both lineages, B/Victoria lineages had a slight preference for MUC5AC-positive cells, and B/Yamagata lineages infected more basal cells. Finally, while both lineages induced a strong interferon response 48 h after infection of hNEC cultures, the B/Victoria lineages showed a much stronger induction of interferon-related signaling pathways compared to B/Yamagata. This demonstrates that the two influenza B virus lineages differ not only in their antigenic structure but also in their ability to induce host innate immune responses.
Keywords: cell tropism; human nasal epithelial cells; influenza B virus; protein immunoassay; viral transcriptomics.
Conflict of interest statement
The authors declare no conflict of interest.
Figures
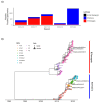
Influenza B clinical incidence and HA phylogenetics. (A) Number of influenza B positive clinical specimens as reported by the Centers for Disease Control and Prevention in the State of Maryland from the ‘WHO NREVSS Public Health Labs’ FluView dataset. Data are summarized by lineage and influenza season. (B) Time-scaled phylogenetic tree of influenza tree of representative HA sequences (n = 282) isolated between 2009 and 2023. Branch tips are colored by lineage with corresponding vaccine strains defined by tip shape. Isolates collected from the Johns Hopkins Hospital network for subsequent characterization in this study are labeled by isolate ID.
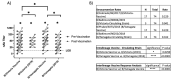
Neutralizing antibody responses to vaccination. (A) Neutralizing antibody titers to pre and post-vaccination sera. Pre- and post-vaccination sera were used to compare the neutralization of B/Yamagata and B/Victoria vaccine strains (B/Phuket/3073/2013 and B/Colorado/06/2017) as well as B/Yamagata and B/Victoria circulating strains for the year 2019–2020 (B/Baltimore/R0250/2018 and B/Baltimore/R0696/2019). (B) Mean difference was calculated, and Sidak’s multiple comparisons test was used to assess significant differences. Statistical differences set at p ≤ 0.05 and indicated by *. **** p < 0.0001.
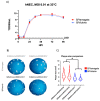
Characterization of viral fitness between B/Yamagata and B/Victoria viruses. (A) Viral fitness assessment on hNEC revealed no significant difference in infectious virus production out to 96 h post-infection of Baltimore clinical isolates. B/Yamagata curves consists of data from B/Baltimore/R0250/2018 and B/Baltimore/R0337/2018 clinical isolates. B/Victoria curves consist of data from B/Baltimore/R0001/2017 and B/Baltimore/R0122/2017. Growth curves completed in two independent experiments, each experiment consisted of three replicate wells. Statistical significance of growth curves calculated on GraphPad Prism using a two-way ANOVA and Tukey’s multiple comparisons. (B) Representative wells shown comparing plaque size from two independent experiments of the two lineages of IBV on MDCK cells. All plaques in experiment wells were counted for analysis. (C) Comparison of plaque sizes formed by two clinical isolates from each IBV lineage. Graphed plaque areas are the combination of two independent experiments. Images of plaques were analyzed with ImageJ. Statistical significance of plaque assays calculated on GraphPad Prism using a two-way ANOVA and Tukey’s multiple comparisons. * denotes statistical significance of p < 0.05.
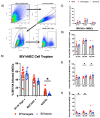
Cell tropism assessment of IBV infection of human nasal cells. (A) Gating strategy of assessing IBV-infected hNECs using flow cytometry. Cells were gated by removing debris, single cells, live cells and IBV HA+. (B) Additional markers of human nasal cells were used to assess cell tropism including BT-IV as a marker of mature ciliated cells, MUC5AC as a marker of mucin-producing cells and NGFR(CD271) as a marker of respiratory basal cells. Statistical Analysis: Percentages of IBV-infected hNECs were statistically compared between two groups using multiple t-tests. Data represent 72 h infection data from two independent runs with three replicates of each virus and two clinical isolates represented within each lineage. Data points shown in the figure represent combined data from two experiments. (C–F) Time course of IBV infection in human nasal epithelial cells. IBV-infected hNECs were identified using HA monoclonal antibodies for IBV HA. The specific cell types infected over the course of infection were identified using antibodies specific for the same cellular targets as shown in panel B. (C) Percent of IBV-infected cells over infection course. (D) Percent of IBV-infected ciliated cells infected over 120 h. (E) Percent of IBV-infected MUC5AC + cells over 120 h. (F) Percent of IBV-infected NGFR+ cells over 120 h. Cell populations were identified with the following gating strategy: excluding debris, single cells, live cells, IBV + cells, specific cellular stain as described. Three replicate wells were completed for this experiment. Data from two clinical isolates included per lineage. Statistical analysis: Percentages of IBV-infected hNECs were statistically compared between two groups using multiple t-tests. * = p < 0.05.
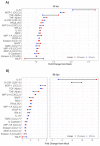
Cytokine expression profiles in the basolateral supernatant of IBV-infected hNEC cultures. (A,B) General profiles of inflammatory signature of IBV-infected hNECs are shown comparing infections with B/Yamagata and B/Victoria clinical isolates. Data represent two independent experiments. For each experiment, three replicate wells were analyzed. Each IBV lineage combines data from two clinical isolates. There were no statistically significant differences between cytokine profiles following infection between B/Yamagata and B/Victoria.
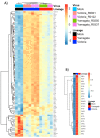
hNEC transcriptional response to B/Victoria and B/Yamagata infection. (A) Heatmap of DEGs with a padj ≤ 0.05 in hNECs (n = 122). (B) Subset the complete heatmap of annotated by the top 24 differentially expressed genes summarized by B/Yamagata and B/Victoria lineages.
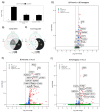
Differentially expressed genes between B/Victoria and B/Yamagata-infected hNECs. Total Differentially expressed genes (DEGs) at threshold cutoffs of a log2 fold change ≥ 0.8 and padj ≤ 0.05. (A) Total up and downregulated genes at threshold cutoffs representative of all DESeq2 design comparisons. Venn diagrams for each design comparison separated by up (B) and down (C) regulated genes. Volcano plots of DEGs at threshold for B/Victoria vs. B/Yamagata, (D) B/Victoria vs. mock (E) and Yamagata vs. mock (F).
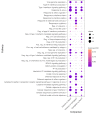
Pathways differentially regulated during B/Victoria and B/Yamgata infection. Pathway enrichment plot representing the top enriched pathways across all lineage comparison using DEseq2 with threshold cutoffs of padj ≤ 0.05 and log2 fold change ≥ 0.8 using gprofiler.
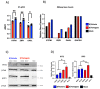
RNAseq validation by RT-qPCR and Western blot. (A) RNAseq validating using RT-qPCR of 5 targets: OASL, IFITM1, RBFOX3, USP17L1 and ZBP1. Change in gene expression was calculated using the 2−(ΔΔCt) method and summarized by lineage. Comparisons were performed by two-tailed t-test using Graphpad Prism. (B) Relative gene expression counts for each validated gene from rlog normalized RNAseq counts. (C) Western blot and relative band intensities of (D) IFIT2 and IFIT3 for each virus by lineage from two independent experiments. Statistical significance of normalized band intensities were calculated on GraphPad Prism using a two-way ANOVA and Tukey’s multiple comparisons. * = p < 0.05 and ** = p < 0.01.
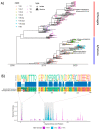
Influenza B NS1 protein has diverged by lineage and HA clade (A) influenza B phylogenetic tree of representative NS1 sequences (n = 283) isolated between 2009 and 2023. NS1 open reading frames were extracted using the NCBI influenza annotation tool (
https://www.ncbi.nlm.nih.gov/genomes/FLU/annotation/(accessed on 14 March 2023)) and aligned using Muscle (v3.8.31) with the PPP algorithm. Time-scaled maximum-likelihood trees were constructed using treetime v0.9.6 and annotated by lineage in R (v4.1.2) using ggtree v3.16. (B) Amino acid alignments were used to calculate Shannon entropy by site using the vegan v2.6-4 and seqinr 4.2-23 packages and visualized using R v4.1.1. IBV NS1 domains were annotated according to PDB: 5DIL and BMRB: 25462. Amino acid site richness and percent identity were visualized using ggmsa 3.16.
Update of
-
Wilson JL, Akin E, Zhou R, Jedlicka A, Dziedzic A, Liu H, Fenstermacher KZ, Rothman R, Pekosz A. Wilson JL, et al. bioRxiv [Preprint]. 2023 Aug 4:2023.08.04.551980. doi: 10.1101/2023.08.04.551980. bioRxiv. 2023. PMID: 37577630 Free PMC article. Updated. Preprint.
Similar articles
-
Rowe T, Davis W, Wentworth DE, Ross T. Rowe T, et al. J Virol. 2024 Feb 20;98(2):e0149423. doi: 10.1128/jvi.01494-23. Epub 2024 Jan 31. J Virol. 2024. PMID: 38294251 Free PMC article.
-
Wilson JL, Akin E, Zhou R, Jedlicka A, Dziedzic A, Liu H, Fenstermacher KZ, Rothman R, Pekosz A. Wilson JL, et al. bioRxiv [Preprint]. 2023 Aug 4:2023.08.04.551980. doi: 10.1101/2023.08.04.551980. bioRxiv. 2023. PMID: 37577630 Free PMC article. Updated. Preprint.
-
Development of high-yield influenza B virus vaccine viruses.
Ping J, Lopes TJ, Neumann G, Kawaoka Y. Ping J, et al. Proc Natl Acad Sci U S A. 2016 Dec 20;113(51):E8296-E8305. doi: 10.1073/pnas.1616530113. Epub 2016 Dec 5. Proc Natl Acad Sci U S A. 2016. PMID: 27930325 Free PMC article.
-
[The Victoria and Yamagata Lineages of Influenza B Viruses, unknown and undervalued].
Reina J. Reina J. Rev Esp Quimioter. 2022 Jun;35(3):231-235. doi: 10.37201/req/159.2021. Epub 2022 Feb 18. Rev Esp Quimioter. 2022. PMID: 35180825 Free PMC article. Review. Spanish.
-
A comprehensive review of influenza B virus, its biological and clinical aspects.
Ashraf MA, Raza MA, Amjad MN, Ud Din G, Yue L, Shen B, Chen L, Dong W, Xu H, Hu Y. Ashraf MA, et al. Front Microbiol. 2024 Sep 4;15:1467029. doi: 10.3389/fmicb.2024.1467029. eCollection 2024. Front Microbiol. 2024. PMID: 39296301 Free PMC article. Review.
Cited by
-
Establishment of a humanized ST6GAL1 mouse model for influenza research.
Chao L, Feng H, Qian G, Limin L, Ziwei L, Shuangshuang L, Xiaoyan L, Yuechao H, Mengjie Y, Yingze Z, Jun L, Xuancheng L, Shuguang D. Chao L, et al. Animal Model Exp Med. 2024 Jun;7(3):337-346. doi: 10.1002/ame2.12449. Epub 2024 Jun 11. Animal Model Exp Med. 2024. PMID: 38859745 Free PMC article.
-
Çalışkan DM, Kumar S, Hinse S, Schughart K, Wiewrodt R, Fischer S, Krueger V, Wiebe K, Barth P, Mellmann A, Ludwig S, Brunotte L. Çalışkan DM, et al. Emerg Microbes Infect. 2024 Dec;13(1):2402868. doi: 10.1080/22221751.2024.2402868. Epub 2024 Sep 23. Emerg Microbes Infect. 2024. PMID: 39248230 Free PMC article.
-
Rowe T, Davis W, Wentworth DE, Ross T. Rowe T, et al. J Virol. 2024 Feb 20;98(2):e0149423. doi: 10.1128/jvi.01494-23. Epub 2024 Jan 31. J Virol. 2024. PMID: 38294251 Free PMC article.
References
-
- CDC . Burden of Influenza. Centers for Disease Control and Prevention; Atlanta, GA, USA: 2022.
-
- Caini S., Huang Q.S., Ciblak M.A., Kusznierz G., Owen R., Wangchuk S., Henriques C.M., Njouom R., Fasce R.A., Yu H., et al. Epidemiological and virological characteristics of influenza B: Results of the Global Influenza B Study. Influenza Other Respir. Viruses. 2015;9((Suppl. 1)):3–12. doi: 10.1111/irv.12319. - DOI - PMC - PubMed
-
- Caini S., Kusznierz G., Garate V.V., Wangchuk S., Thapa B., de Paula Júnior F.J., Ferreira de Almeida W.A., Njouom R., Fasce R.A., Bustos P., et al. The Epidemiological Signature of Influenza B Virus and Its B/Victoria and B/Yamagata Lineages in the 21st Century. PLoS ONE. 2019;14:e0222381. doi: 10.1371/journal.pone.0222381. - DOI - PMC - PubMed
MeSH terms
Substances
Grants and funding
LinkOut - more resources
Full Text Sources
Medical