Harvesting of aerial humidity with natural hygroscopic salt excretions - PubMed
- ️Sun Jan 01 2023
Harvesting of aerial humidity with natural hygroscopic salt excretions
Marieh B Al-Handawi et al. Proc Natl Acad Sci U S A. 2023.
Abstract
Plants and animals that thrive in arid regions utilize the diurnal changes in environmental temperature and humidity to optimize their water budget by combining water-harvesting mechanisms and morphophysiological traits. The Athel tamarisk (Tamarix aphylla) is a halophytic desert shrub that survives in arid, hypersaline conditions by excreting concentrated solutions of ions as droplets on its surface that crystallize into salt crystals and fall off the branches. Here, we describe the crystallization on the surface of the plant and explore the effects of external conditions such as diurnal changes in humidity and temperature. The salt mixtures contain at least ten common minerals, with NaCl and CaSO4·2H2O being the major products, SiO2 and CaCO3 main sand contaminants, and Li2SO4, CaSO4, KCl, K2Ca(SO4)2·H2O, CaMg(CO3)2 and AlNaSi3O8 present in smaller amounts. In natural conditions, the hanging or sitting droplets remain firmly attached to the surface, with an average adhesion force of 275 ± 3.5 µN measured for pure water. Rather than using morphological features of the surface, the droplets adhere by chemical interactions, predominantly by hydrogen bonding. Increasing ion concentration slightly increases the contact angle on the hydrophobic cuticle, thereby lowering surface wettability. Small amounts of lithium sulfate and possibly other hygroscopic salts result in strong hygroscopicity and propensity for deliquescence of the salt mixture overnight. Within a broader context, this natural mechanism for humidity harvesting that uses environmentally benign salts as moisture adsorbents could provide a bioinspired approach that complements the currently available water collection or cloud-seeding technologies.
Keywords: biomineralization; crystallization; deliquescence; extremopohiles; water collection.
Conflict of interest statement
The authors declare no competing interest.
Figures
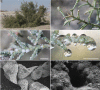
Appearance of the shrub, branches, and surfaces of T. aphylla. (A) A photograph of T. aphylla located across the coastal sabkha of the United Arab Emirates. (B) A close-up view of the branches. (C) A branch recorded in the late morning (11 a.m.) was encrusted with salt crystals. (D) A branch recorded in the early morning (8 a.m.) showed condensed water droplets. (E and F) Scanning electron microscopic images of the surfaces of leaves with salt crystals (E) and a zoomed image of a single salt gland (F). The length of the scale bars in panels B, C, D, E, and F are 5 mm, 3 mm, 3 mm, 1 mm, and 50 µm, respectively.
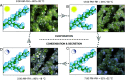
A summary of the water collection and salt crystallization over the diurnal cycle of T. aphylla. (A) The plant condenses water on its surface in the early morning when the humidity is high, and the temperature is low (9 a.m., 60% RH, 20 °C). (B) The water starts to evaporate, and the salts crystallize on the surface of the branch around midday, when the humidity is low and the temperature is high (12 p.m. noon, 35% RH, 30 °C). (C) During daytime, the water evaporates, resulting in the formation of salt crystals (7 p.m., 52% RH, 22 °C). (D) Overnight, when the humidity is high, the crystals adsorb water and dissolve (2 a.m., 80% RH, 18 °C).
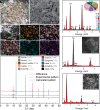
Characterization of the salts crystallized from the excretion of T. aphylla. (A) Optical image of the salt harvested from the branches. (B) Scanning electron micrograph (SEM) of the salt crystals, (C–H and I) SEM-EDX mapping and spectra of the plant salt. (J) Synchrotron PXRD pattern of the salt excreted by the plant and collected on May 2021 (sample ID: PS-4) (K and L) SEM images and EDX analysis of a cubic-shaped crystal (K) and a flower-shaped crystal (L). Scale bars for panels A and B are 5 mm and 500 μm, respectively, for C–H are 500 μm, and for the insets in panels K and L are 200 μm and 50 μm, respectively.
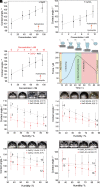
Dependence of the contact angle of salt solutions on salt concentration, humidity, and temperature. (A–C) The contact angle as measured for solutions of NaCl (A), CaSO4·2H2O (B), and NaCl mixed with CaSO4·2H2O (C) at different concentrations on a silicon wafer coated with epicuticular wax extracted from the leaves. (D) Adhesion force curves of a water droplet on the wax-coated silicon wafer. (i–v) schematic representation of the behavior of a water droplet on the surface of wax: (i) attachment of the droplet to the surface, (ii–iv) stretching of the droplet and the retraction of the stage, and (v) detachment of the droplet from the surface. (E–H) Contact angle measurements performed at different concentrations of NaCl [35 mM (E), 95 mM (F)] and CaSO4·2H2O [3.5 mM (G), 9.5 mM (H)], at two different temperatures, 21.5 °C (black) and 35 °C (red). The measurements were performed on a silicon wafer coated with the extracted epicuticular wax from the leaves. All measurements were performed under 35%, 45%, 55%, 65%, 75%, and 85% relative humidity (RH). Images of the droplets under various conditions are shown above each panel.
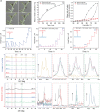
Water-collection and water-resorption ability of a freshly cleaved branch and the salt assessed by vapor sorption, calorimetry, and PXRD upon deliquescence. (A–D) Snapshots showing salt crystals attached to a branch deliquesce over time under high humidity (80%, 35 °C). (E) Mass of the water collected by the branch over time, together with a control branch where the salt crystals have been removed. (F) Ratio of the mass of water collected to mass of the sample as a function of thumidity. (G–I) Change in heat flow as a result in change of the relative humidity between 35 to 85% as a function of time of the plant salt (G), a model salt mixture composed of 80% NaCl and 20% CaSO4·2H2O (H), and a model mixture composed of 80% NaCl, 19% CaSO4·2H2O and 1% Li2SO4 (I). (J–N). Changes in the NaCl and CaSO4·2H2O diffraction peak intensities during the deliquescence and dissolution of the crystals induced by increase in humidity. (O–S) Dehydration and recrystallization of salts as humidity decreases from 85 to 15%. The sample in Fig. 5E was collected in September 2019. The analysis in Fig. 5F was performed on a different branch collected on May 2020. The analysis in Fig. 5G was performed on salt samples collected in January 2021 (PS-2). Fig. 5J shows the analysis of salt and branch collected in November 2020 (PS-1).
Similar articles
-
Zhang H, Gu W, Li YJ, Tang M. Zhang H, et al. J Environ Sci (China). 2020 Sep;95:65-72. doi: 10.1016/j.jes.2020.03.046. Epub 2020 Apr 9. J Environ Sci (China). 2020. PMID: 32653194
-
Coopman RE, Nguyen HT, Mencuccini M, Oliveira RS, Sack L, Lovelock CE, Ball MC. Coopman RE, et al. New Phytol. 2021 Aug;231(4):1401-1414. doi: 10.1111/nph.17461. Epub 2021 Jun 12. New Phytol. 2021. PMID: 33983649
-
Hygroscopic salts and the potential for life on Mars.
Davila AF, Duport LG, Melchiorri R, Jänchen J, Valea S, de Los Rios A, Fairén AG, Möhlmann D, McKay CP, Ascaso C, Wierzchos J. Davila AF, et al. Astrobiology. 2010 Jul-Aug;10(6):617-28. doi: 10.1089/ast.2009.0421. Astrobiology. 2010. PMID: 20735252
-
Nielsen LS, Šantl-Temkiv T, Palomeque Sánchez M, Massling A, Ward JC, Jensen PB, Boesen T, Petters M, Finster K, Bilde M, Rosati B. Nielsen LS, et al. Environ Sci Technol. 2024 Oct 29;58(43):19211-19221. doi: 10.1021/acs.est.4c01817. Epub 2024 Oct 19. Environ Sci Technol. 2024. PMID: 39425695
-
Sun K, Nguyen CV, Nguyen NN, Nguyen AV. Sun K, et al. Adv Colloid Interface Sci. 2022 Nov;309:102775. doi: 10.1016/j.cis.2022.102775. Epub 2022 Sep 16. Adv Colloid Interface Sci. 2022. PMID: 36152375 Review.
References
-
- UNICEF, Progress on Sanitation and Drinking Water: 2015 Update and MDG Assessment (World Health Organization, 2015).
-
- UNCCD, Desertification: The Invisible Frontline (United Nations Conventions to Combat Desertification, 2014).
-
- Eller C. B., Lima A. L., Oliveira R. S., Foliar Uptake of fog water and transport belowground alleviates drought effects in the cloud forest tree species, Drimys brasiliensis (Winteraceae). New Phytol. 199, 151–162 (2013). - PubMed
-
- Martin C. E., von Willert D. J., Leaf epidermal hydathodes and the ecophysiological consequences of foliar water uptake in species of Crassula from the Namib Desert in Southern Africa. Plant Biol. 2, 229–242 (2000).
-
- Wang X., Xiao H., Ren J., Cheng Y., Yang Q., An ultrasonic humidification fluorescent tracing method for detecting unsaturated atmospheric water absorption by the aerial parts of desert plants. J. Arid Land 8, 272–283 (2016).
Grants and funding
LinkOut - more resources
Full Text Sources