ATM inhibition blocks glucose metabolism and amplifies the sensitivity of resistant lung cancer cell lines to oncogene driver inhibitors - PubMed
- ️Sun Jan 01 2023
ATM inhibition blocks glucose metabolism and amplifies the sensitivity of resistant lung cancer cell lines to oncogene driver inhibitors
Cristina Terlizzi et al. Cancer Metab. 2023.
Abstract
Background: ATM is a multifunctional serine/threonine kinase that in addition to its well-established role in DNA repair mechanisms is involved in a number of signaling pathways including regulation of oxidative stress response and metabolic diversion of glucose through the pentose phosphate pathway. Oncogene-driven tumorigenesis often implies the metabolic switch from oxidative phosphorylation to glycolysis which provides metabolic intermediates to sustain cell proliferation. The aim of our study is to elucidate the role of ATM in the regulation of glucose metabolism in oncogene-driven cancer cells and to test whether ATM may be a suitable target for anticancer therapy.
Methods: Two oncogene-driven NSCLC cell lines, namely H1975 and H1993 cells, were treated with ATM inhibitor, KU55933, alone or in combination with oncogene driver inhibitors, WZ4002 or crizotinib. Key glycolytic enzymes, mitochondrial complex subunits (OXPHOS), cyclin D1, and apoptotic markers were analyzed by Western blotting. Drug-induced toxicity was assessed by MTS assay using stand-alone or combined treatment with KU55933 and driver inhibitors. Glucose consumption, pyruvate, citrate, and succinate levels were also analyzed in response to KU55933 treatment. Both cell lines were transfected with ATM-targeted siRNA or non-targeting siRNA and then exposed to treatment with driver inhibitors.
Results: ATM inhibition deregulates and inhibits glucose metabolism by reducing HKII, p-PKM2Tyr105, p-PKM2Ser37, E1α subunit of pyruvate dehydrogenase complex, and all subunits of mitochondrial complexes except ATP synthase. Accordingly, glucose uptake and pyruvate concentrations were reduced in response to ATM inhibition, whereas citrate and succinate levels were increased in both cell lines indicating the supply of alternative metabolic substrates. Silencing of ATM resulted in similar changes in glycolytic cascade and OXPHOS levels. Furthermore, the driver inhibitors amplified the effects of ATM downregulation on glucose metabolism, and the combined treatment with ATM inhibitors enhanced the cytotoxic effect of driver inhibitors alone by increasing the apoptotic response.
Conclusions: Inhibition of ATM reduced both glycolytic enzymes and OXPHOS levels in oncogene-driven cancer cells and enhanced apoptosis induced by driver inhibitors thus highlighting the possibility to use ATM and the driver inhibitors in combined regimens of anticancer therapy in vivo.
Keywords: ATM; Anticancer therapy; Glycolysis; OXPHOS; Oncogene-driver inhibitors.
© 2023. The Author(s).
Conflict of interest statement
The authors declare no competing interests.
Figures
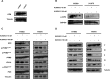
Effects of ATM inhibition on glycolysis and OXPHOS in oncogene-driven NSCLC H1993 and H1975 cells. A Baseline levels of ATM protein in both cell lines. B Inhibition of ATM phosphorylation after 48-h exposure to 10-nM and 100-nM of KU55933. C Levels of HKII, phospho-PKM2Tyr105, phospho-PKM2Ser37, PKM2, LDH-A, phospho-PDHSer293, and PDH in basal conditions and after 48-h exposure to 10-nM and 100-nM of KU55933. D Levels of OXPHOS in basal conditions and after 48-h exposure to 10-nM and 100-nM of KU55933. Vinculin, GAPDH, and tubulin serve as equal loading controls
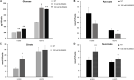
Levels of extracellular glucose and intracellular metabolic intermediates in response to ATM inhibition. A Levels of residual glucose in culture media of H1993 and H1975 cells exposed to 10-nM and 100-nM of KU55933 for 48 h. Levels of intracellular B pyruvate, C citrate, and D succinate in H1993 and H1975 cells exposed to 100-nM of KU55933 for 48 h. Symbol * indicates a p-value < 0.05, symbol ** indicates a p-value < 0.01, symbol *** indicates a p-value < 0.001 versus NT
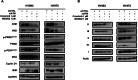
Effects of ATM silencing in oncogene-driven H1993 and H1975 cells. A Levels of HKII, phospho-PKM2Tyr105, PKM2, phospho-PDHSer293, PDH, cyclin D1, and BIM in H1993 and H1975 cells transfected with ATM-targeted small interfering RNA or siRNA control and exposed to crizotinib or WZ4002 for 48 h. B Levels of OXPHOS in H1993 and H1975 cells transfected with ATM-targeted small interfering RNA or siRNA control and exposed to crizotinib or WZ4002 for 48 h. GAPDH and actin serve as equal loading controls
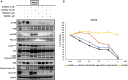
Combined treatment with KU55933 and WZ4002 in H1975 cells. A Levels of p-ATM, ATM, p-EGFR, PGC-1α, p-p53, p53, PARP and cleaved PARP, BIM, cleaved-lamin A/C, and cyclin D1 in H1975 treated with KU55933 (10-nM and 100-nM), WZ4002 (0.5 μM and 1 μM), and their combination for 48 h. B Cell viability assay performed in H1975 cells exposed to increasing concentration of KU55933 (0.01–5 μM) or WZ4002 (0.01–5 μM) alone and to combination treatment with a fixed concentration of KU55933 (10-nM and 100-nM) plus increasing doses of WZ4002 (0.01–5 μM). Tubulin serves as equal loading control
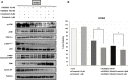
Combined treatment with KU55933 and crizotinib in H993 cells. A Levels of p-ATM, ATM, PGC-1α, p-p53.Ser15, p53, PARP and cleaved PARP, BIM, and cyclin D1 in H1993 treated with KU55933 (10- nM and 100-nM), crizotinib (0.5 μM and 1 μM), and their combination for 48 h. B Cell viability assay performed in H1993 cells exposed to KU55933 (100-nM), crizotinib (0.5 μM–1 μM), and their combination. Tubulin serves as equal loading control. Symbol * indicates a p-value < 0.05. Symbol *** indicates a p-value < 0.001
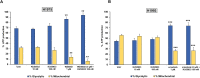
Percentages of glycolytic and mitochondrial ATP production in untreated and treated H1975 and H1993 cells. A H1975 cells were treated for 48 h with 10-nM and 100-nM KU55933, 1-μM WZ4002, and a combination of 100-nM KU55933 with 0.5-μM WZ4002. B H1993 cells were treated for 48 h with 10-nM and 100-nM KU55933, 1-μM crizotinib, and a combination of 100-nM KU55933 with 0.5-μM crizotinib. Compared to untreated cells, the symbol ** indicates p < 0.01, whereas *** indicates p < 0.001
Similar articles
-
De Rosa V, Iommelli F, Monti M, Fonti R, Votta G, Stoppelli MP, Del Vecchio S. De Rosa V, et al. Clin Cancer Res. 2015 Nov 15;21(22):5110-20. doi: 10.1158/1078-0432.CCR-15-0375. Epub 2015 Jul 27. Clin Cancer Res. 2015. PMID: 26216352
-
De Rosa V, Iommelli F, Terlizzi C, Leggiero E, Camerlingo R, Altobelli GG, Fonti R, Pastore L, Del Vecchio S. De Rosa V, et al. Cancers (Basel). 2021 Aug 17;13(16):4133. doi: 10.3390/cancers13164133. Cancers (Basel). 2021. PMID: 34439291 Free PMC article.
-
De Rosa V, Monti M, Terlizzi C, Fonti R, Del Vecchio S, Iommelli F. De Rosa V, et al. Int J Mol Sci. 2019 Jun 27;20(13):3134. doi: 10.3390/ijms20133134. Int J Mol Sci. 2019. PMID: 31252559 Free PMC article.
-
Metabolic phenotype of bladder cancer.
Massari F, Ciccarese C, Santoni M, Iacovelli R, Mazzucchelli R, Piva F, Scarpelli M, Berardi R, Tortora G, Lopez-Beltran A, Cheng L, Montironi R. Massari F, et al. Cancer Treat Rev. 2016 Apr;45:46-57. doi: 10.1016/j.ctrv.2016.03.005. Epub 2016 Mar 8. Cancer Treat Rev. 2016. PMID: 26975021 Review.
-
Nonhormonal therapy for endometriosis based on energy metabolism regulation.
Kobayashi H, Shigetomi H, Imanaka S. Kobayashi H, et al. Reprod Fertil. 2021 Nov 25;2(4):C42-C57. doi: 10.1530/RAF-21-0053. eCollection 2021 Dec. Reprod Fertil. 2021. PMID: 35118411 Free PMC article. Review.
Cited by
-
Sheikhhossein HH, Iommelli F, Di Pietro N, Curia MC, Piattelli A, Palumbo R, Roviello GN, De Rosa V. Sheikhhossein HH, et al. Vaccines (Basel). 2024 May 9;12(5):519. doi: 10.3390/vaccines12050519. Vaccines (Basel). 2024. PMID: 38793770 Free PMC article. Review.
References
LinkOut - more resources
Full Text Sources
Research Materials
Miscellaneous