IFN-γ lowers tumor growth by increasing glycolysis and lactate production in a nitric oxide-dependent manner: implications for cancer immunotherapy - PubMed
- ️Sun Jan 01 2023
IFN-γ lowers tumor growth by increasing glycolysis and lactate production in a nitric oxide-dependent manner: implications for cancer immunotherapy
Avik Chattopadhyay et al. Front Immunol. 2023.
Abstract
Introduction: Interferon-gamma (IFN-γ), the sole member of the type-II interferon family, is well known to protect the host from infectious diseases as well as mount anti-tumor responses. The amounts of IFN-γ in the tumor microenvironment determine the host responses against tumors; however, several tumors employ evasive strategies by responding to low IFN-γ signaling.
Methods: In this study, the response of various tumor cell lines to IFN-γ was studied in vitro.
Results: IFN-γ-activation increases glycolytic flux and reduces mitochondrial function in a nitric oxide (NO)- and reactive oxygen species (ROS)-dependent manner in the H6 hepatoma tumor cell line. The higher glycolysis further fueled NO and ROS production, indicating a reciprocal regulation. These processes are accompanied by Hypoxia inducing factor (HIF)-1α stabilization and HIF-1α-dependent augmentation of the glycolytic flux. The IFN-γ enhancement of lactate production also occurred in other NO-producing cell lines: RAW 264.7 monocyte/macrophage and Renca renal adenocarcinoma. However, two other tumor cell lines, CT26 colon carcinoma and B16F10 melanoma, did not produce NO and lactate upon IFN-γ-activation. HIF-1α stabilization upon IFN-γ-activation led to lower cell growth of B16F10 but not CT26 cells. Importantly, the IFN-γ-activation of both CT26 and B16F10 cells demonstrated significant cellular growth reduction upon metabolic rewiring by exogenous administration of potassium lactate.
Discussion: Clinical studies have shown the crucial roles of IFN-γ for successful cancer immunotherapies involving checkpoint inhibitors and chimeric antigen receptor T cells. The positive implications of this study on the metabolic modulation of IFN-γ activation on heterogeneous tumor cells are discussed.
Keywords: HIF-1 alpha; interferon-gamma; lactate; nitric oxide; tumor.
Copyright © 2023 Chattopadhyay, Jagdish, Karhale, Ramteke, Zaib and Nandi.
Conflict of interest statement
The authors declare that the research was conducted in the absence of any commercial or financial relationships that could be construed as a potential conflict of interest.
Figures
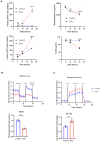
IFN-γ-activation of H6 cells increases glycolytic flux and compromises mitochondrial function. H6 hepatoma cells were seeded in a 6-well plate and treated with 10 U/mL IFN-γ for the indicated time points. The cell numbers were counted, and the cell-free supernatant was collected and assayed for nitrite, residual glucose, and lactate. The nitrite and lactate levels were normalized to the percent cell number (A). The Seahorse XF analysis of the H6 cells was performed post-IFN-γ-activation for 24 hours. The pharmacological modulators were sequentially injected as indicated. The mitostress test with the OCR from the 4th measurement (B) and the glycolysis stress test with the ECAR from the 3rd measurement (C) are shown. Statistical analyses in this study were conducted utilizing two-way ANOVA in conjunction with Tukey’s multiple comparisons tests (A) and unpaired t-tests (A–C). The notation “ns” indicates non-significant differences, while (*), (**), (***), and (****) denote statistical significance levels of p < 0.05, p < 0.01, p < 0.001, and p < 0.0001, respectively, for comparisons between the groups specified. Each data point is a representative observation derived from an independent experiment. The data are expressed as the mean ± standard deviation based on 3 to 5 independent experiments.
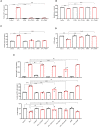
IFN-γ-activated augmentation of glycolytic flux is NO and ROS-dependent in H6 cells. H6 cells were treated with 10 U/ml IFN-γ and incubated alone or with pharmacological inhibitors of NOS enzymes (LNMA at 200 uM and 1400W at 6 uM) for 24 hours to inhibit NO biosynthesis. The nitrite levels were measured from the cell-free supernatants and normalized to the percent cell number (A). The residual glucose concentrations in the cell-free supernatant were measured after 24 hours of IFN-γ-activation of the H6 cells (B). The lactate concentrations were measured in the same cell-free supernatant and normalized to percent cell number (C). The cell numbers were counted and represented as the percent cell number (D). The H6 cells were treated with 10 U/ml IFN-γ and incubated alone or with the peroxynitrite quencher (FeTPPS at 50 uM), inhibitors of ROS production (PEG–CAT at 100 U/ml and PEG–SOD at 50 U/ml) and soluble guanylate cyclase inhibitor ODQ at 20 uM for 24 hours. The cell numbers were counted and represented as the percent cell number. The nitrite and lactate concentrations were measured in the cell-free supernatant and normalized to percent cell number. (E). Statistical analyses in this study were conducted utilizing ordinary one-way ANOVA in conjunction with Sidak’s multiple comparisons tests. The notation “ns” indicates non-significant differences, while (*), (***), and (****) denote statistical significance levels of p < 0.05, p < 0.001, and p < 0.0001, respectively, for comparisons between the groups specified. Each data point is a representative observation derived from an independent experiment. The data are expressed as the mean ± standard deviation based on 3 to 5 independent experiments.
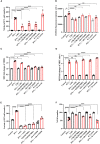
IFN-γ-mediated enhanced glycolysis fuels IFN-γ signaling responses by increasing NO and decreasing cell number. H6 cells were treated with 10 U/ml IFN-γ and incubated alone or with pharmacological modulators of glycolysis (2-DG, a hexokinase inhibitor at 2.5 mM; GSK2837808A, a lactate dehydrogenase inhibitor at 125 nM; AR-C155858, a monocarboxylate transporter inhibitor at 6 nM and K-lactate as a metabolic reprogramming agent at 10 mM). The cell-free supernatants were collected after 24 hours, and the level of nitrite and lactate were estimated (A, E). The cell numbers were counted to derive the percent cell number (F). The levels of nitrite and lactate were normalized to the percent cell number. The intracellular ROS was measured using a Tecan microplate reader from 0.1 million cells/well upon DCFDA staining for 30 minutes (B). The IFN-γ-activated H6 cells were assayed for glucose uptake and surface expression of MHC class 1 using flow cytometry in the presence of the glycolysis modulators. The cells were incubated with 100 μM 2-NBDG for 30 minutes, and flow cytometric analysis of glucose uptake was performed (C). The cells were stained with an antibody to MHC class 1, and flow cytometric analysis of the surface expression of MHC Class 1 was performed (D). Statistical analyses in this study were conducted utilizing ordinary one-way ANOVA in conjunction with Sidak’s multiple comparisons tests. The notation “ns” indicates non-significant differences, while (*), (**), (***), and (****) denote statistical significance levels of p < 0.05, p < 0.01, p < 0.001, and p < 0.0001, respectively, for comparisons between the groups specified. Each data point is a representative observation derived from an independent experiment. The data are expressed as the mean ± standard deviation based on 3 independent experiments.
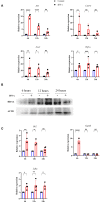
IFN-γ-activation stabilizes HIF-1α and induces several HIF-1α-responsive glycolytic genes. H6 cells were treated with 10 U/ml IFN-γ in a 6-well plate for the indicated time points, and total RNA was extracted. RT-qPCR was performed to quantify the relative expression of Irf1, Nos2, Cd274, and Hif1a (A). The cells were lysed at the indicated time, and immunoblot was performed to quantify the HIF-1α intracellular protein amounts. β-Actin was used as the loading control (B). The relative mRNA expression of several HIF-1α target genes, such as Hk2, Gapdh, and Ldha, was also quantified using RT-qPCR (C). Statistical analyses in this study were conducted utilizing two-way ANOVA in conjunction with Tukey’s multiple comparisons tests. The notation “ns” indicates non-significant differences, while (*), (**), (***), and (****) denote statistical significance levels of p < 0.05, p < 0.01, p < 0.001, and p < 0.0001, respectively, for comparisons between the groups specified. Each data point is a representative observation derived from an independent experiment. The data are expressed as the mean ± standard deviation based on 3 to 4 independent experiments.
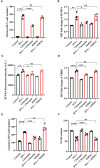
IFN-γ-activated augmentation of glycolytic flux is dependent on HIF-1α in H6 cells. H6 cells were treated with 10 U/ml IFN-γ for 24 hours. The IFN-γ-activated cells were incubated alone or with pharmacological modulators of HIF-1α function (Chetomin, an inhibitor of HIF-1α at 12.5 nM, and DMOG, a stabilizer of HIF-1α at 0.5 uM). The cell-free supernatant was collected and the levels of nitrite and lactate were estimated in the cell-free supernatant. The nitrite and lactate levels are normalized to percent cell number (A, E). The IFN-γ-activated H6 cells were assayed for intracellular ROS by incubating the cells with 10 μM DCFDA for 30 minutes. The analyses of intracellular ROS were performed using flow cytometry (B) and Tecan microplate reader-based estimation (C). The cells were incubated with 100 μM 2-NBDG for 30 minutes, and flow cytometric analysis of glucose uptake was performed (D). The cell number was counted to derive the percent cell number (F). Statistical analyses in this study were conducted utilizing ordinary one-way ANOVA in conjunction with Sidak’s multiple comparisons tests. The notation “ns” indicates non-significant differences, while (*), (**), (***), and (****) denote statistical significance levels of p < 0.05, p < 0.01, p < 0.001, and p < 0.0001, respectively, for comparisons between the groups specified. Each data point is a representative observation derived from an independent experiment. The data are expressed as the mean ± standard deviation based on 3 to 5 independent experiments.
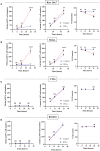
Only IFN-γ-activated NO-producing tumor cells increase lactate production and reduce cell number. Raw 264.7 (A), Renca (B), CT26 (C), and B16F10 (D) cell lines were treated with 10 U/mL IFN-γ in a 24-well plate. The cell culture medium was collected kinetically. The levels of nitrite and lactate were measured from the cell-free supernatant. The cell number was counted to derive the percent cell number. The statistical analyses were performed using two-way ANOVA with Tukey’s multiple comparisons tests for comparing nitrite and lactate levels and unpaired t-test for comparing the percent cell number. The notation “ns” indicates non-significant differences, while (*), (**), and (****) denote statistical significance levels of p < 0.05, p < 0.01, and p < 0.0001, respectively, for comparisons between the groups specified. Each data point is a representative observation derived from an independent experiment. The data are expressed as the mean ± standard deviation based on 3 to 5 independent experiments.
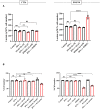
K-lactate in the presence of IFN-γ lowers the growth of non-NO-producing cell lines, CT26 and B16F10 cells. CT26 and B16F10 cells were treated with 10 U/ml IFN-γ and incubated alone or with pharmacological modulators (SNAP, an NO donor at 25 μM; DMOG, a stabilizer of HIF-1α at 0.5 μM and K-lactate as a metabolic reprogramming agent at 10 mM) for 24 hours. The level of lactate was measured from the cell-free supernatant. The lactate level was normalized to the percent cell number (A). The cell number was counted to derive the percent cell number (B). Statistical analyses in this study were conducted utilizing ordinary one-way ANOVA in conjunction with Sidak’s multiple comparisons tests. The notation “ns” indicates non-significant differences, while (**), (***), and (****) denote statistical significance levels of p < 0.01, p < 0.001, and p < 0.0001, respectively, for comparisons between the groups specified. Each data point is a representative observation derived from an independent experiment. The data are expressed as the mean ± standard deviation based on 3 independent experiments.
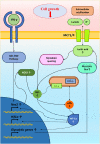
A graphical illustration of IFN-γ-mediated enhanced NO and ROS increasing glycolysis and reducing tumor growth. IFN-γ-activation of H6 cells induced NO production and NO-mediated ROS generation. NO and ROS impair mitochondrial membrane potential and may reduce mitochondrial O2 consumption. NO, ROS and damaged mitochondrial function might stabilize HIF-1α levels. HIF-1α enhanced the glycolytic flux upon IFN-γ-activation, possibly through increasing glycolytic gene expression. The enhanced glycolytic flux increased extracellular acidification and lactate accumulation. Furthermore, the heightened glycolysis and lactate reciprocally promoted the IFN-γ-induced NO and ROS production, reducing tumor cell growth. Solid arrows indicate connections with evidence. Dashed arrows indicate probable regulations.
Similar articles
-
Leung E, Cairns RA, Chaudary N, Vellanki RN, Kalliomaki T, Moriyama EH, Mujcic H, Wilson BC, Wouters BG, Hill R, Milosevic M. Leung E, et al. BMC Cancer. 2017 Jun 15;17(1):418. doi: 10.1186/s12885-017-3402-6. BMC Cancer. 2017. PMID: 28619042 Free PMC article.
-
Martinez-Outschoorn U, Sotgia F, Lisanti MP. Martinez-Outschoorn U, et al. Semin Oncol. 2014 Apr;41(2):195-216. doi: 10.1053/j.seminoncol.2014.03.002. Epub 2014 Mar 5. Semin Oncol. 2014. PMID: 24787293 Review.
-
Zeng L, Zhou HY, Tang NN, Zhang WF, He GJ, Hao B, Feng YD, Zhu H. Zeng L, et al. World J Gastroenterol. 2016 May 28;22(20):4868-80. doi: 10.3748/wjg.v22.i20.4868. World J Gastroenterol. 2016. PMID: 27239113 Free PMC article.
-
Van Dis E, Fox DM, Morrison HM, Fines DM, Babirye JP, McCann LH, Rawal S, Cox JS, Stanley SA. Van Dis E, et al. PLoS Pathog. 2022 Jul 25;18(7):e1010721. doi: 10.1371/journal.ppat.1010721. eCollection 2022 Jul. PLoS Pathog. 2022. PMID: 35877763 Free PMC article.
-
Marín-Hernández A, Gallardo-Pérez JC, Ralph SJ, Rodríguez-Enríquez S, Moreno-Sánchez R. Marín-Hernández A, et al. Mini Rev Med Chem. 2009 Aug;9(9):1084-101. doi: 10.2174/138955709788922610. Mini Rev Med Chem. 2009. PMID: 19689405 Review.
Cited by
-
Toledo B, Zhu Chen L, Paniagua-Sancho M, Marchal JA, Perán M, Giovannetti E. Toledo B, et al. J Hematol Oncol. 2024 Jun 11;17(1):44. doi: 10.1186/s13045-024-01559-0. J Hematol Oncol. 2024. PMID: 38863020 Free PMC article. Review.
-
Differential Anti-Inflammatory Effects of Electrostimulation in a Standardized Setting.
Di Pietro B, Villata S, Dal Monego S, Degasperi M, Ghini V, Guarnieri T, Plaksienko A, Liu Y, Pecchioli V, Manni L, Tenori L, Licastro D, Angelini C, Napione L, Frascella F, Nardini C. Di Pietro B, et al. Int J Mol Sci. 2024 Sep 11;25(18):9808. doi: 10.3390/ijms25189808. Int J Mol Sci. 2024. PMID: 39337300 Free PMC article.
References
Publication types
MeSH terms
Substances
Grants and funding
The author(s) declare financial support was received for the research, authorship, and/or publication of this article. This study was funded by SERB grant CRG/2021/004284, core grants from IISc and the DBT-IISc partnership program. In addition, we thank the DST-FIST grant to the Department of Biochemistry, IISc for the infrastructural support.
LinkOut - more resources
Full Text Sources