Enhanced catalytic performance of penicillin G acylase by covalent immobilization onto functionally-modified magnetic Ni0.4Cu0.5Zn0.1Fe2O4 nanoparticles - PubMed
- ️Mon Jan 01 2024
Enhanced catalytic performance of penicillin G acylase by covalent immobilization onto functionally-modified magnetic Ni0.4Cu0.5Zn0.1Fe2O4 nanoparticles
Zhixiang Lv et al. PLoS One. 2024.
Abstract
With the emergence of penicillin resistance, the development of novel antibiotics has become an urgent necessity. Semi-synthetic penicillin has emerged as a promising alternative to traditional penicillin. The demand for the crucial intermediate, 6-aminopicillanic acid (6-APA), is on the rise. Enzyme catalysis is the primary method employed for its production. However, due to certain limitations, the strategy of enzyme immobilization has also gained prominence. The magnetic Ni0.4Cu0.5Zn0.1Fe2O4 nanoparticles were successfully prepared by a rapid-combustion method. Sodium silicate was used to modify the surface of the Ni0.4Cu0.5Zn0.1Fe2O4 nanoparticles to obtain silica-coated nanoparticles (Ni0.4Cu0.5Zn0.1Fe2O4-SiO2). Subsequently, in order to better crosslink PGA, the nanoparticles were modified again with glutaraldehyde to obtain glutaraldehyde crosslinked Ni0.4Cu0.5Zn0.1Fe2O4-SiO2-GA nanoparticles which could immobilize the PGA. The structure of the PGA protein was analyzed by the PyMol program and the immobilization strategy was determined. The conditions of PGA immobilization were investigated, including immobilization time and PGA concentration. Finally, the enzymological properties of the immobilized and free PGA were compared. The optimum catalytic pH of immobilized and free PGA was 8.0, and the optimum catalytic temperature of immobilized PGA was 50°C, 5°C higher than that of free PGA. Immobilized PGA in a certain pH and temperature range showed better catalytic stability. Vmax and Km of immobilized PGA were 0.3727 μmol·min-1 and 0.0436 mol·L-1, and the corresponding free PGA were 0.7325 μmol·min-1 and 0.0227 mol·L-1. After five cycles, the immobilized enzyme activity was still higher than 25%.
Copyright: © 2024 Lv et al. This is an open access article distributed under the terms of the Creative Commons Attribution License, which permits unrestricted use, distribution, and reproduction in any medium, provided the original author and source are credited.
Conflict of interest statement
The authors have declared that no competing interests exist.
Figures
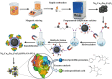
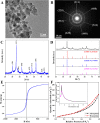
Transmission electron microscopy (TEM) image (A), selected area electron diffraction (SAED) spectrum (B), X-ray diffraction (XRD) pattern (C), XRD standard card (D), vibrating sample magnetometry (VSM) curve (E), and Nitrogen adsorption isotherm and pore distribution map (F) of the magnetic Ni0.4Cu0.5Zn0.1Fe2O4 nanoparticles prepared at 400°C for 2.0 h with a heating rate of 3°C·min-1.
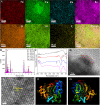
The EDS maps (A) and EDS spectrogram (B) of Ni0.4Cu0.5Zn0.1Fe2O4@SiO2-GA, FTIR spectra (C) of Ni0.4Cu0.5Zn0.1Fe2O4 nanoparticles (a), Ni0.4Cu0.5Zn0.1Fe2O4@SiO2 (b), Ni0.4Cu0.5Zn0.1Fe2O4@SiO2-GA (c) and Ni0.4Cu0.5Zn0.1Fe2O4@SiO2-GA-PGA (d), the HRTEM gram (D) and Lattice fringe picture (E) of Ni0.4Cu0.5Zn0.1Fe2O4@SiO2-GA, the docking pattern of penicillin G and PGA (F), and the molecular model of PGA (G).
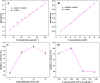
The standard curve of protein (A) and 6-APA (B), the effects of immobilization time (C) and PGA concentration (D) on the catalytic activity of the immobilized enzyme.
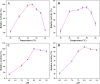
The catalytic capacity of free PGA (A) and immobilized PGA (B) at different temperatures, the effects of different pH on the activity of free PGA (C) and immobilized PGA (D).
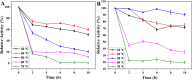
Thermal stability of free PGA (A) and immobilized PGA (B).
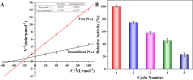
The Michaelis constant of free and immobilized PGA (A), and the Repetitive availability of immobilized PGA (B).
Similar articles
-
Lv Z, Yu Q, Wang Z, Liu R. Lv Z, et al. J Microbiol Biotechnol. 2019 Jun 28;29(6):913-922. doi: 10.4014/jmb.1903.03017. J Microbiol Biotechnol. 2019. PMID: 31154745
-
Liu R, Chen D, Fu H, Lv P, Zhang D, He Y. Liu R, et al. J Nanosci Nanotechnol. 2017 Feb;17(2):893-99. doi: 10.1166/jnn.2017.12694. J Nanosci Nanotechnol. 2017. PMID: 29671470
-
Penicillin G acylase-based stationary phases: analytical applications.
Calleri E, Temporini C, Massolini G, Caccialanza G. Calleri E, et al. J Pharm Biomed Anal. 2004 Apr 16;35(2):243-58. doi: 10.1016/S0731-7085(03)00587-9. J Pharm Biomed Anal. 2004. PMID: 15063459 Review.
-
Berillo D, Malika T, Baimakhanova BB, Sadanov AK, Berezin VE, Trenozhnikova LP, Baimakhanova GB, Amangeldi AA, Kerimzhanova B. Berillo D, et al. Gels. 2024 Oct 10;10(10):646. doi: 10.3390/gels10100646. Gels. 2024. PMID: 39451299 Free PMC article. Review.
References
-
- Arteche-Eguizabal L, Corcuera-Martínez De Tobillas I, Melgosa-Latorre F, Domingo-Echaburu S, Urrutia-Losada A, Eguiluz-Pinedo A, et al.. Multidisciplinary Collaboration for the Optimization of Antibiotic Prescription: Analysis of Clinical Cases of Pneumonia between Emergency, Internal Medicine, and Pharmacy Services. Antibiotics. 2022;11: 1336. doi: 10.3390/antibiotics11101336 - DOI - PMC - PubMed
MeSH terms
Substances
Grants and funding
The Jiangsu Provincial Postgraduate Scientific Practice and Innovation Project (Grant No. SJCX21_1722). The Sichuan Science and Technology Program (Grant No. 2020YJ0492). The funders had no role in study design, data collection and analysis, decision to publish, or preparation of the manuscript.
LinkOut - more resources
Full Text Sources
Miscellaneous