Precise genome-editing in human diseases: mechanisms, strategies and applications - PubMed
- ️Mon Jan 01 2024
Review
Precise genome-editing in human diseases: mechanisms, strategies and applications
Yanjiang Zheng et al. Signal Transduct Target Ther. 2024.
Abstract
Precise genome-editing platforms are versatile tools for generating specific, site-directed DNA insertions, deletions, and substitutions. The continuous enhancement of these tools has led to a revolution in the life sciences, which promises to deliver novel therapies for genetic disease. Precise genome-editing can be traced back to the 1950s with the discovery of DNA's double-helix and, after 70 years of development, has evolved from crude in vitro applications to a wide range of sophisticated capabilities, including in vivo applications. Nonetheless, precise genome-editing faces constraints such as modest efficiency, delivery challenges, and off-target effects. In this review, we explore precise genome-editing, with a focus on introduction of the landmark events in its history, various platforms, delivery systems, and applications. First, we discuss the landmark events in the history of precise genome-editing. Second, we describe the current state of precise genome-editing strategies and explain how these techniques offer unprecedented precision and versatility for modifying the human genome. Third, we introduce the current delivery systems used to deploy precise genome-editing components through DNA, RNA, and RNPs. Finally, we summarize the current applications of precise genome-editing in labeling endogenous genes, screening genetic variants, molecular recording, generating disease models, and gene therapy, including ex vivo therapy and in vivo therapy, and discuss potential future advances.
© 2024. The Author(s).
Conflict of interest statement
The authors declare no competing interests.
Figures
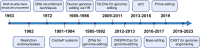
Timeline of the development of precise genome-editing tools. Key milestones in precise genome-editing are indicated. HR Homologous recombination, ZFNs Zinc-finger nucleases, TALENs Transcription activator-like effector nucleases, HITI Homology-independent targeted integration, CAST CRISPR-associated transposase. This figure was produced using BioRender.com
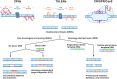
Precise genome-editing with site specific nucleases. ZFNs create double-strand breaks (DSBs) using the FokI restriction enzyme paired with specific zinc-finger DNA-binding domains. TALENs induce DSBs using the FokI restriction enzyme in conjunction with specific TALE DNA-binding domains. Cas9 nuclease, targeted by guide RNAs, creates DSBs using two distinct domains of nuclease. Genome-editing utilizing nucleases relies on two primary DNA repair pathways. The first pathway consists of end-joining mechanisms, which can be divided into classical nonhomologous end-joining (c-NHEJ), which can be used to produce targeted semi-random indels, and homology-independent target integration (HITI), which can be used to insert an exogenous sequence at a desired genomic target in the absence of homology arms. The second major repair mechanism is homology-directed repair (HDR), which primarily occurs in dividing cells, and can be used to create precise targeted edits via a single-strand or double-strand DNA donor template. ZFNs Zinc-finger nucleases, TALENs Transcription activator-like effector nucleases. This figure was produced using BioRender.com
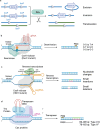
Strategies of non HDR-mediated precise genome-editing. a Cre/loxP system, consisting of Cre recombinase and loxP sites, facilitates DNA recombination through excision (removing a DNA segment between loxP sites in the same orientation), inversion (flipping a segment between loxP sites in opposite orientations), and translocation exchanging segments between two loxP sites in the same orientation on different DNA strands. b Base editing involves the introduction of C•G-to-T•A or C•G-to-G•C point mutations using cytosine base editors (CBEs), which employ Cas9 nickase or dCas9 fused to cytidine deaminase. Additionally, A•T-to-C•G point mutations can be reversed through adenine base editors (ABEs), utilizing a fusion of dCas9 or Cas9 nickase and evolved TadA* deoxyadenosine deaminase. c Prime editors comprise a Cas9 nickase domain fused to a reverse transcriptase domain. A prime editing guide RNA (pegRNA), engineered for specificity, directs the prime editor to its target on genomic DNA, including the desired edit within an extension. Following nicking the PAM-containing strand, the freed genomic DNA 3’ end engages in a primer–template complex with the pegRNA extension. Subsequently, the reverse transcriptase domain copies the template from the pegRNA extension into the genomic DNA directly, facilitating the addition of point mutations, small deletions, or small insertions at the target locus. d CAST combines Cas proteins with transposase-associated components. Transposase proteins (Tns) bind to transposon DNA, while Cas proteins are guided to the target locus in a PAM-dependent, RNA-directed manner. This localization facilitates transposon DNA integration at the target site, with each Cas-transposase complex having a specific guide RNA length and a preferred integration distance 3’ of the PAM. This figure was produced using BioRender.com
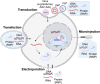
Delivery strategies for precise genome-editing reagents. Precise genome-editing components encompass a variety of forms, including DNA, RNA, and protein complexes such as ribonucleoproteins (RNPs). DNA is commonly delivered through microinjection or electroporation of plasmids, as well as viral vectors such as lentivirus, adeno-associated virus (AAV), and adenovirus (AdV). RNA can be introduced through microinjection or electroporation of RNPs, or via carriers like lipid nanoparticles (LNPs) and virus-like particles (VLPs). Proteins, specifically RNPs, are typically delivered through microinjection or electroporation, or using carriers like LNPs and VLPs. This figure was produced using BioRender.com
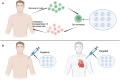
Ex vivo and in vivo precise genome-editing therapy. a In ex vivo editing therapy, cells are harvested from the patient, modified, and subsequently reintroduced. b For in vivo systemic therapy (on the left), universal delivery agents are employed, capable of targeting a broad range of tissue types. For targeted in vivo therapy (on the right), targeted intervention can be accomplished by directly injecting viral vectors into the affected tissue or through systemic administration of vectors with an innate affinity for particular tissues, such as the heart, liver, or skeletal muscle. This figure was produced using BioRender.com
Similar articles
-
Delivery Aspects of CRISPR/Cas for in Vivo Genome Editing.
Wilbie D, Walther J, Mastrobattista E. Wilbie D, et al. Acc Chem Res. 2019 Jun 18;52(6):1555-1564. doi: 10.1021/acs.accounts.9b00106. Epub 2019 May 17. Acc Chem Res. 2019. PMID: 31099553 Free PMC article.
-
Precision genome editing in the CRISPR era.
Salsman J, Dellaire G. Salsman J, et al. Biochem Cell Biol. 2017 Apr;95(2):187-201. doi: 10.1139/bcb-2016-0137. Epub 2016 Sep 29. Biochem Cell Biol. 2017. PMID: 28177771 Review.
-
Development of CRISPR technology for precise single-base genome editing: a brief review.
Lee HK, Oh Y, Hong J, Lee SH, Hur JK. Lee HK, et al. BMB Rep. 2021 Feb;54(2):98-105. doi: 10.5483/BMBRep.2021.54.2.217. BMB Rep. 2021. PMID: 33298245 Free PMC article. Review.
-
Gene Therapy with CRISPR/Cas9 Coming to Age for HIV Cure.
Soriano V. Soriano V. AIDS Rev. 2017 Oct-Dec;19(3):167-172. AIDS Rev. 2017. PMID: 29019352
-
Wei T, Cheng Q, Farbiak L, Anderson DG, Langer R, Siegwart DJ. Wei T, et al. ACS Nano. 2020 Aug 25;14(8):9243-9262. doi: 10.1021/acsnano.0c04707. Epub 2020 Jul 22. ACS Nano. 2020. PMID: 32697075 Free PMC article.
Cited by
-
Gene therapy for the leukodystrophies: From preclinical animal studies to clinical trials.
Metovic J, Li Y, Gong Y, Eichler F. Metovic J, et al. Neurotherapeutics. 2024 Jul;21(4):e00443. doi: 10.1016/j.neurot.2024.e00443. Epub 2024 Sep 13. Neurotherapeutics. 2024. PMID: 39276676 Free PMC article. Review.
-
Reporter Alleles in hiPSCs: Visual Cues on Development and Disease.
Cotta GC, Teixeira Dos Santos RC, Costa GMJ, Lacerda SMDSN. Cotta GC, et al. Int J Mol Sci. 2024 Oct 13;25(20):11009. doi: 10.3390/ijms252011009. Int J Mol Sci. 2024. PMID: 39456792 Free PMC article. Review.
References
Publication types
MeSH terms
Substances
LinkOut - more resources
Full Text Sources
Other Literature Sources