Timed receptor tyrosine kinase signaling couples the central and a peripheral circadian clock in Drosophila - PubMed
- ️Mon Jan 01 2024
Timed receptor tyrosine kinase signaling couples the central and a peripheral circadian clock in Drosophila
Javier Cavieres-Lepe et al. Proc Natl Acad Sci U S A. 2024.
Abstract
Circadian clocks impose daily periodicities to behavior, physiology, and metabolism. This control is mediated by a central clock and by peripheral clocks, which are synchronized to provide the organism with a unified time through mechanisms that are not fully understood. Here, we characterized in Drosophila the cellular and molecular mechanisms involved in coupling the central clock and the peripheral clock located in the prothoracic gland (PG), which together control the circadian rhythm of emergence of adult flies. The time signal from central clock neurons is transmitted via small neuropeptide F (sNPF) to neurons that produce the neuropeptide Prothoracicotropic Hormone (PTTH), which is then translated into daily oscillations of Ca2+ concentration and PTTH levels. PTTH signaling is required at the end of metamorphosis and transmits time information to the PG through changes in the expression of the PTTH receptor tyrosine kinase (RTK), TORSO, and of ERK phosphorylation, a key component of PTTH transduction. In addition to PTTH, we demonstrate that signaling mediated by other RTKs contributes to the rhythmicity of emergence. Interestingly, the ligand to one of these receptors (Pvf2) plays an autocrine role in the PG, which may explain why both central brain and PG clocks are required for the circadian gating of emergence. Our findings show that the coupling between the central and the PG clock is unexpectedly complex and involves several RTKs that act in concert and could serve as a paradigm to understand how circadian clocks are coordinated.
Keywords: PTTH; circadian rhythms; eclosion; neuropeptide; prothoracic gland.
Conflict of interest statement
Competing interests statement:The authors declare no competing interest.
Figures
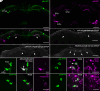
Connectivity between PDF-expressing sLNv clock neurons and PTTHn. (A–A″) Cell bodies and projections from PTTHn in the superior protocerebrum (A). PDF-expressing sLNv clock neurons project to the superior protocerebrum (A′) in close proximity to the projections of PTTHn (arrows in merged image, A″). (B) syb-GRASP reconstruction between PTTHn and PDF-expressing sLNv clock neurons produced only a faint and sparse reconstruction of GFP along the primary neurite (arrows), which did not include small branches in the contact area between PTTHn and sLNv located in the superior protocerebral region. (B′) syb-GRASP reconstruction between PTTHn and DN1p clock neurons labeled by Clk4.1 M-LexA produced a varicose branched signal. (B″) syb-GRASP reconstruction between PTTHn and DN1a, DN2, LNd, and LPN clock neurons labeled by R43D05-LexA (24) produced a varicose branched signal. In comparison (B–B″), the sparse vs. varicose syb-GRASP signals suggest that PTTHn receive non-synaptic input from the sLNvs, and synaptic input from other clock neurons. (C–C″) BAcTrace-labeling showing that all PDF-expressing sLNv but not the large lLNv synapse onto the PTTHn. Whereas pdf-LexA drives GFP expression in the sLNv and lLNv (C), the BAcTrace signal (C′) was only detected in the sLNv (C″). (D–D″) trans-Tango MkII labeling showed that all PTTHn are downstream to the sLNv since the positive trans-Tango MkII signal was visible in PTTHn present in each hemisphere (D′). (E–E″). A PDF receptor-specific intragenic driver line (E″) did not label the PTTHn (E) in pharate adults, indicating that the PDF receptor is not expressed (E′). Asterisks mark the position of the PTTHn. (F–F″). By contrast, an sNPF receptor-specific intragenic driver line (F″), labeled the PTTHn (F) in pharate adults (F′), indicating sNPF receptor expression. (Scale bars: A and B: 50 μm; C–F: 20 μm.)
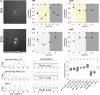
Ca+2 signaling in PTTHn is relevant to the circadian control of adult emergence. (A and B) Representative images of GCaMP fluorescence in PTTHn in the brains of WPP examined at ZT4 (A) and ZT12 (B). (C–F) Levels of GCaMP fluorescence in PTTHn of wild-type (WT) animals at different times of day under LD (C) and DD conditions (E), and in the soma of PTTHn of per[0] mutants under LD (D) and DD (F) conditions. Different letters indicate statistically different groups (ANOVA with Tukey's post hoc test). Between 8 and 10 animals were analyzed for each timepoint. ZT: zeitgeber time. CT: Circadian time. (G–I) Records showing the time course of emergence of a single population of flies under DD conditions (Left) and corresponding autocorrelation analysis (Right) of populations bearing a knockdown in PTTHn of Ryanodine Receptor (RyR) (G), SERCA (H), and in corresponding controls. (I) Periodicity (p, in hours) and associated rhythmicity index (RI) are indicated. (J) Average RI values from knockdown in PTTHn of mediators of Ca+2 signaling. The dashed line at RI = 0.1 indicates cutoff below which records are considered arrhythmic. Different letters indicate statistically different groups (P < 0.05; one-way ANOVA, Tukey’s post hoc multiple comparison analyses; see
SI Appendix, Table S1and
Dataset S1for all statistical analyses of emergence rhythmicity and of expression data, respectively).
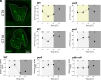
Clock control of PTTH/TORSO axis. (A and B) Pattern of PTTH-immunoreactivity of terminals of PTTHn on the PG of WPP animals at CT6 (A) and CT18 (B). The white line delimits the PG. (C–F) Average number of PTTH-immunoreactive boutons showing above-threshold intensity on the PG at different times of day in WT (C and E) and in per[0] mutants (D and F) under LD (C and D) and DD (E and F) conditions. Eight to ten brains were analyzed per time-point. (G–I) Relative expression of mRNA levels of torso in PGs isolated from WPP stage WT (G), per[0] (H), and ptth null mutant (I) animals under DD conditions. Different letters indicate statistically different groups (P < 0.05; one-way ANOVA, Tukey’s post hoc multiple comparison analyses; see
Dataset S1for all statistical analyses of expression data).
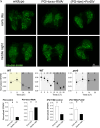
ERK phosphorylation in the PG shows daily variations that are dependent on torso and Pvr. (A–F) ERK-SPARK signal in whole mount PGs (phm>ERK-SPARK) of WT, PG>torso-RNAi, and (PG+tim)>PvrDN WPP animals at CT0-2 (early subjective day) and CT18-20 (middle of subjective night). (G–I) Average of normalized ERK-SPARK signal in the PG at different times of day in WT PG under LD (G), DD (H) conditions, and in per[0] mutants (under DD condition; I). (J and K) Average normalized ERK-SPARK signal in (J) PG>w and PG>torso-RNAi and (K) (PG+tim)>w and (PG+tim)>PvrDN animals, at CT0-2 (early subjective day) and CT18-20 (middle of subjective night). Eight to ten brains were analyzed per time-point. Different letters indicate statistically different groups (P < 0.05; one-way ANOVA, Tukey’s post hoc multiple comparison analyses; see
Dataset S1for all statistical analyses of expression data).
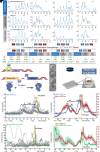
PTTHn activity is required during the end of pupal development. (A) Record of the time course of emergence in ptth>tubP-Gal80ts, Kir2.1 flies with PTTHn conditionally silenced at 29 °C during larval (L), entire pupal (EP+LP), early pupal (EP), and late pupal stages (LP), and in corresponding controls. (B) RI, Lomb–Scargle (LS), and eJTK_Cycle values of experiments shown in (A). Rhythmic records are coded in green, arrhythmic ones in red. Raising the temperature to 29 °C during metamorphosis accelerates development, and when this period included the late pupal stage (LP), it causes emergence to occur over a short period of time. This renders autocorrelation inappropriate for rhythmicity analysis. Hence, values are given for LS and eJTK_Cycle analysis only. In addition, rhythmic records show peaks followed by valleys that are separated by ~24 h (arrows); see Materials and Methods for further details. (C–F) In vivo imaging of ARG-Luc signal in PTTHn and PG prior to emergence. (C–D′) Schematic representation of the ARG-Luc system and in vivo imaging setup. (D) Images of intact animals captured at different stages: pharate [1]; eclosing [2]; eclosed; prior to [3], and after [4], wing expansion. (E) ARG-Luc activity of PTTHn for 14 individual flies plotted relative to the time of emergence (yellow line); the blue line corresponds to the average trace. (F) Average ARG-Luc activity for the PTTHn (blue) and for the PG (red, n = 22). (G) ARG-Luc activity of PGs expressing the dominant negative form of Pvr (PvrDN) plotted relative to eclosion (yellow line) (n = 19). The mean activity is plotted in green. (H) Mean activity of the PG expressing PvrDN [green, same curve as in (G)] is plotted against the mean of normal PG activity [same curve as in (F)]. Error bars indicate SEM. Expression of PvrDN in the PG strongly reduced the amplitude of the ARG-Luc signal (note different scales for Y-axes) without significantly affecting its timecourse. Error bars indicate SEM. For PTTHn, 21 animals were recorded, but the records of only 14 were included in E and F; the remaining 7 animals were excluded because their signal did not change during the recording period, most likely because they were either located too far from the sensor or were not correctly oriented. The peaks immediately following eclosion in (E–H) are likely artifacts due to higher photon yields once flies have left their absorbing puparium.

Alk and Pvr contribute to the circadian rhythmicity of adult emergence. (A–H) Records showing the time course of emergence under DD (Left) and corresponding autocorrelation analysis (Right) of a single population of flies expressing: in the PG, a dominant negative form of Pvr (B), Alk RNAi (C), simultaneous knockdown of Pvr and Alk receptors (D), Pvf2 RNAi (G) and Pvf3 RNAi (H); and expressing in PTTHn: Jeb RNAi (E) and Pvf3 RNAi (F). Periodicity (p, in hours) and associated RI are indicated. (I–K) Average RI values for results shown in (A–H) and their respective controls; dashed line indicates cutoff below which records are considered arrhythmic. Different letters indicate statistically significant differences. NP423 is a strong driver that includes PTTHn. *P < 0.05; one-way ANOVA, Tukey post hoc analysis;
SI Appendix, Table S1for all statistical analyses of emergence rhythmicity.
Similar articles
-
Central and peripheral clocks are coupled by a neuropeptide pathway in Drosophila.
Selcho M, Millán C, Palacios-Muñoz A, Ruf F, Ubillo L, Chen J, Bergmann G, Ito C, Silva V, Wegener C, Ewer J. Selcho M, et al. Nat Commun. 2017 May 30;8:15563. doi: 10.1038/ncomms15563. Nat Commun. 2017. PMID: 28555616 Free PMC article.
-
The Circadian Clock Is a Key Driver of Steroid Hormone Production in Drosophila.
Di Cara F, King-Jones K. Di Cara F, et al. Curr Biol. 2016 Sep 26;26(18):2469-2477. doi: 10.1016/j.cub.2016.07.004. Epub 2016 Aug 18. Curr Biol. 2016. PMID: 27546572
-
The circadian clock gates Drosophila adult emergence by controlling the timecourse of metamorphosis.
Mark B, Bustos-González L, Cascallares G, Conejera F, Ewer J. Mark B, et al. Proc Natl Acad Sci U S A. 2021 Jul 6;118(27):e2023249118. doi: 10.1073/pnas.2023249118. Proc Natl Acad Sci U S A. 2021. PMID: 34183412 Free PMC article.
-
Circadian orchestration of developmental hormones in the insect, Rhodnius prolixus.
Steel CG, Vafopoulou X. Steel CG, et al. Comp Biochem Physiol A Mol Integr Physiol. 2006 Jul;144(3):351-64. doi: 10.1016/j.cbpa.2006.02.018. Epub 2006 Mar 6. Comp Biochem Physiol A Mol Integr Physiol. 2006. PMID: 16702005 Review.
-
Takeda M, Suzuki T. Takeda M, et al. Front Physiol. 2022 Jun 22;13:867621. doi: 10.3389/fphys.2022.867621. eCollection 2022. Front Physiol. 2022. PMID: 35812309 Free PMC article. Review.
References
-
- Buijs F. N., et al. , The circadian system: A regulatory feedback network of periphery and brain. Physiology (Bethesda) 31, 170–181 (2016). - PubMed
-
- Harder L., Oster H., The tissue clock network: Driver and gatekeeper of circadian physiology: Circadian rhythms are integrated outputs of central and peripheral tissue clocks interacting in a complex manner—From drivers to gatekeepers. Bioessays 42, e1900158 (2020). - PubMed
-
- Pilorz V., Helfrich-Forster C., Oster H., The role of the circadian clock system in physiology. Pflugers Arch. 470, 227–239 (2018). - PubMed
MeSH terms
Substances
Grants and funding
- 1221270/ANID | Fondo Nacional de Desarrollo Científico y Tecnológico (FONDECYT)
- 21180133/Agencia Nacional de Investigación y Desarrollo (ANID)
- P09-022-F/Agencia Nacional de Investigación y Desarrollo (ANID)
- DFG WE2652/7-1/Deutsche Forschungsgemeinschaft (DFG)
- STA 421/8-1/Deutsche Forschungsgemeinschaft (DFG)
LinkOut - more resources
Full Text Sources
Molecular Biology Databases
Miscellaneous