The scheme, and regulative mechanism of pyroptosis, ferroptosis, and necroptosis in radiation injury - PubMed
- ️Mon Jan 01 2024
Review
. 2024 Mar 3;20(5):1871-1883.
doi: 10.7150/ijbs.91112. eCollection 2024.
Affiliations
- PMID: 38481804
- PMCID: PMC10929204
- DOI: 10.7150/ijbs.91112
Review
The scheme, and regulative mechanism of pyroptosis, ferroptosis, and necroptosis in radiation injury
Jiaoyang Ning et al. Int J Biol Sci. 2024.
Abstract
Radiotherapy (RT) stands as the primary treatment for tumors, but it inevitably causes damage to normal cells. Consequently, radiation injury is a crucial consideration for radiation oncologists during therapy planning. Cell death including apoptosis, autophagy, pyroptosis, ferroptosis, and necroptosis play significant roles in tumor treatment. While previous studies elucidated the induction of apoptosis and autophagy by ionizing radiation (IR), recent attention has shifted to pyroptosis, ferroptosis, and necroptosis, revealing their effects induced by IR. This review aims to summarize the strategies employed by IR, either alone or in combination therapy, to induce pyroptosis, ferroptosis, and necroptosis in radiation injury. Furthermore, we explore their effects and molecular pathways, shedding light on their roles in radiation injury. Finally, we summarize the regulative agents for these three types of cell death and their mechanisms. In summary, optimizing radiation dose, dose rate, and combined treatment plans to minimize radiation damage and enhance the killing effect of RT is a key focus.
Keywords: Ferroptosis; Necroptosis; Pyroptosis; Radiation injury; Regulative mechanism.
© The author(s).
Conflict of interest statement
Competing Interests: The authors have declared that no competing interest exists.
Figures
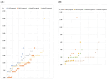
IR dose for pyroptosis- and ferroptosis-related radiation injury. (A) Total dose (B) Dose rate.
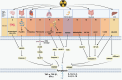
Regulative mechanism of inhibitors for treating pyroptosis-related radiation injury. (A) The feverfew plant extract ACT001 inhibits NLRP3 and reduces the expression of IL-6, TNF-α, IL-1β, and GSDMD, thereby inhibiting pyroptosis and radiation-induced lung injury. (B) I-Histidine, secreted by intestinal flora, mitigates radiation-induced cardiopulmonary injury by inhibiting pyroptosis. (C) Bacterial-derived FlaAN/C inhibits ROS, NLRP3, and caspase-1, thus attenuating intestinal cells pyroptosis and the release of inflammation-related cytokines, including IL-1β, IL-18, IL-8, IL-6, and TNF-α. (D) rMnSOD inhibits radiation-induced liver damage by inactivating caspase-1, aSMase, and nSMases. (E) Micheliolide inhibits the NLRP3/caspase-1 axis, attenuating pyroptosis and the release of cytokines including IL-1β, IL-18, TGF-β1, TNF-α, and IFN-γ, which ultimately promots mice survival after radiation exposure. (F) p-Coumaric acid inactivates caspase-1, NLRP3, and AIM2 to inhibit pyroptosis and radiation-induced lung injury. (G-H) VX-765 and the NF-κB inhibitor PDTC inhibit caspase-1 to alleviate radiation-induced intestinal damage. (I) 5-AED upregulates the expression of NF-κB to mitigate AIM2-driven pyroptosis and radiation-induced intestinal injury. (J) NaNO3 inhibits pyroptosis and acinar cells damage by downregulating the expression of NLPR3, ASC, GSDMD, IL-18, thus inhibiting pyroptosis. (K) Pulmozyme inhibits double-strand DNA damage and the the cGAS/STING/NLRP3 axis activation and the release of IL-1β, IL-18, protecting lung tissue from radiation damage. (L) Mesenchymal stem cells inhibit NLRP3 and caspase-1 to alleviate radiation-induced brain damage.
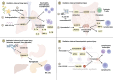
The role of immune regulation in pyroptosis- and ferroptosis-related radiation damage. (A) Radiation-induced lung injury. (Aa-b) Andrographolide and miR-223-3p inhibit macrophage pyroptosis, the release of IL-1α, IL-1β, IL-6, IL-18, TNF-α and radiation-induced lung injury through the AIM2/caspase-1 and NLRP3/caspase-1 axes, respectively. (Ac) RDN inhibits the AIM2/caspase-1 axis, reducing the recruitment of immune cells such as macrophages, neutrophils, and T lymphocytes, and mitigating radiation-induced lung injury. (B) Radiation-induced intestinal injury. (Ba) FLASH-RT inhibits the cGAS-STING pathway, attenuating GSDME-induced pyroptosis and cytotoxic T cells infiltration, ultimately protecting intestinal cells from radiation damage. (Bb) Liproxstatin-1 upregulates LPCAT3 and ALOX15 expression and inhibits mitochondrial damage, ultimately preventing ferroptosis, the release of IL-6, IL-17, TNF-α and lymphocytes infiltration. (C) Radiation-induced multi-organ (intestine, stomach, liver and pancreas) injury. Inhibition of pyroptosis by regulating the GSDME/caspase-3 axis blocks NK cells recruitment, mitigating radiation-induced multi-organ damage. (D) Radiation-induced hematopoietic system injury. (Da-b) Ferrostatin-1 and LDN 193189 inhibit iron metabolism to protect the hematopoietic system from radiation damage.
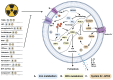
Regulative mechanism of inhibitors for treating ferroptosis-related radiation damage. Radiotherapy can stimulate (A) iron metabolism and (B) ROS metabolism while inhibiting (C) the system Xc--GPX4 axis, ultimately inducing ferroptosis. This process is suppressed by several inhibitors. (A) Iron metabolism: NCOA4 promotes ferritinophagy, inhibiting the expression of iron storage-related proteins such as FTH and FTL, while suppressing FPN1 and enhancing TRF1, thereby synergistically promoting iron metabolism. Activation of HO-1, facilitated by NRF2, further inhibits iron metabolism. Dysregulated iron metabolism leads to ROS accumulation. (B) ROS metabolism: NRF2 upregulates NQO1, which counteracts ROS levels elevated by ACSL4 and induces increased PTGS2 expression. Elevated ROS levels promote lipid peroxidation, triggering ferroptosis. (C) System Xc--GPX4: SLC38A9 activates mTOR, inhibiting system Xc- comprised of SLC7A11 and SLC3A2. Cystine influx via system Xc- activates the GSH/GPX4 axis, inhibiting ROS accumulation. ACSL4 indirectly inhibits system Xc- and GPX4, thereby promoting ROS accumulation. Ferroptosis induction leads to the release of cytokines, including IL-1β, IL-6, IL-10, TNF-α, and TGF-β1.
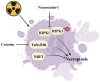
Regulative mechanism of inhibitors for treating necroptosis-related radiation damage. Crocetin and necrostatin-1 inhibit necroptosis by suppressing the radiation-induced upregulation of Tnfrsf10b and RIPK1/p-RIPK3 expression respectively.
Similar articles
-
Zhou P, Zhang S, Wang M, Zhou J. Zhou P, et al. Biomolecules. 2023 May 11;13(5):820. doi: 10.3390/biom13050820. Biomolecules. 2023. PMID: 37238692 Free PMC article. Review.
-
Wang Y, Wang Y, Pan J, Gan L, Xue J. Wang Y, et al. Radiother Oncol. 2023 Jul;184:109689. doi: 10.1016/j.radonc.2023.109689. Epub 2023 May 6. Radiother Oncol. 2023. PMID: 37150447 Review.
-
Ferroptosis, necroptosis, and pyroptosis in anticancer immunity.
Tang R, Xu J, Zhang B, Liu J, Liang C, Hua J, Meng Q, Yu X, Shi S. Tang R, et al. J Hematol Oncol. 2020 Aug 10;13(1):110. doi: 10.1186/s13045-020-00946-7. J Hematol Oncol. 2020. PMID: 32778143 Free PMC article. Review.
-
Regulated Necrotic Cell Death in Alternative Tumor Therapeutic Strategies.
Woo Y, Lee HJ, Jung YM, Jung YJ. Woo Y, et al. Cells. 2020 Dec 17;9(12):2709. doi: 10.3390/cells9122709. Cells. 2020. PMID: 33348858 Free PMC article. Review.
-
Tong X, Tang R, Xiao M, Xu J, Wang W, Zhang B, Liu J, Yu X, Shi S. Tong X, et al. J Hematol Oncol. 2022 Dec 8;15(1):174. doi: 10.1186/s13045-022-01392-3. J Hematol Oncol. 2022. PMID: 36482419 Free PMC article. Review.
Cited by
-
Jin Y, Zhang H, Zhou M, Zhang S, Guo M. Jin Y, et al. Int J Gen Med. 2024 Nov 6;17:5069-5084. doi: 10.2147/IJGM.S485153. eCollection 2024. Int J Gen Med. 2024. PMID: 39526065 Free PMC article.
-
Ferroptosis: emerging roles in lung cancer and potential implications in biological compounds.
Liang Q, Wang Y, Li Y, Wang J, Liu C, Li Y. Liang Q, et al. Front Pharmacol. 2024 May 9;15:1374182. doi: 10.3389/fphar.2024.1374182. eCollection 2024. Front Pharmacol. 2024. PMID: 38783959 Free PMC article. Review.
-
Mechanisms of radiation-induced tissue damage and response.
Zhou L, Zhu J, Liu Y, Zhou PK, Gu Y. Zhou L, et al. MedComm (2020). 2024 Sep 20;5(10):e725. doi: 10.1002/mco2.725. eCollection 2024 Oct. MedComm (2020). 2024. PMID: 39309694 Free PMC article. Review.
-
Pretreatment can alleviate programmed cell death in mesenchymal stem cells.
Wan XX, Hu XM, Zhang Q, Xiong K. Wan XX, et al. World J Stem Cells. 2024 Aug 26;16(8):773-779. doi: 10.4252/wjsc.v16.i8.773. World J Stem Cells. 2024. PMID: 39219726 Free PMC article.
References
-
- Smith BD, Haffty BG, Wilson LD, Smith GL, Patel AN, Buchholz TA. The future of radiation oncology in the United States from 2010 to 2020: will supply keep pace with demand? Journal of clinical oncology: official journal of the American Society of Clinical Oncology. 2010;28:5160–5. - PubMed
-
- Huang Y, Wang H, Luo G, Zhang Y, Wang L, Li K. A systematic review and network meta-analysis of neoadjuvant therapy combined with surgery for patients with resectable esophageal squamous cell carcinoma. International journal of surgery (London, England) 2017;38:41–7. - PubMed
-
- Tallet AV, Dhermain F, Le Rhun E, Noël G, Kirova YM. Combined irradiation and targeted therapy or immune checkpoint blockade in brain metastases: toxicities and efficacy. Annals of oncology: official journal of the European Society for Medical Oncology. 2017;28:2962–76. - PubMed
-
- Pitroda SP, Chmura SJ, Weichselbaum RR. Integration of radiotherapy and immunotherapy for treatment of oligometastases. The Lancet Oncology. 2019;20:e434–e42. - PubMed
Publication types
MeSH terms
LinkOut - more resources
Full Text Sources