Intrinsically disordered proteins: Ensembles at the limits of Anfinsen's dogma - PubMed
- ️Sat Jan 01 2022
Review
. 2022 Mar 17;3(1):011306.
doi: 10.1063/5.0080512. eCollection 2022 Mar.
Vitor B P Leite 2 , Susmita Roy 3 , Supriyo Bhattacharyya 4 , Atish Mohanty 1 , Srisairam Achuthan 5 , Divyoj Singh 6 , Rajeswari Appadurai 7 , Govindan Rangarajan 8 , Keith Weninger 9 , John Orban, Anand Srivastava 7 , Mohit Kumar Jolly 6 , Jose N Onuchic 10 , Vladimir N Uversky, Ravi Salgia 1
Affiliations
- PMID: 38505224
- PMCID: PMC10903413
- DOI: 10.1063/5.0080512
Review
Intrinsically disordered proteins: Ensembles at the limits of Anfinsen's dogma
Prakash Kulkarni et al. Biophys Rev (Melville). 2022.
Abstract
Intrinsically disordered proteins (IDPs) are proteins that lack rigid 3D structure. Hence, they are often misconceived to present a challenge to Anfinsen's dogma. However, IDPs exist as ensembles that sample a quasi-continuum of rapidly interconverting conformations and, as such, may represent proteins at the extreme limit of the Anfinsen postulate. IDPs play important biological roles and are key components of the cellular protein interaction network (PIN). Many IDPs can interconvert between disordered and ordered states as they bind to appropriate partners. Conformational dynamics of IDPs contribute to conformational noise in the cell. Thus, the dysregulation of IDPs contributes to increased noise and "promiscuous" interactions. This leads to PIN rewiring to output an appropriate response underscoring the critical role of IDPs in cellular decision making. Nonetheless, IDPs are not easily tractable experimentally. Furthermore, in the absence of a reference conformation, discerning the energy landscape representation of the weakly funneled IDPs in terms of reaction coordinates is challenging. To understand conformational dynamics in real time and decipher how IDPs recognize multiple binding partners with high specificity, several sophisticated knowledge-based and physics-based in silico sampling techniques have been developed. Here, using specific examples, we highlight recent advances in energy landscape visualization and molecular dynamics simulations to discern conformational dynamics and discuss how the conformational preferences of IDPs modulate their function, especially in phenotypic switching. Finally, we discuss recent progress in identifying small molecules targeting IDPs underscoring the potential therapeutic value of IDPs. Understanding structure and function of IDPs can not only provide new insight on cellular decision making but may also help to refine and extend Anfinsen's structure/function paradigm.
© 2022 Author(s).
Conflict of interest statement
The authors declare no conflict of interest.
Figures
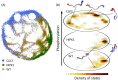
The different PAGE4 ensembles represented in a single conformational phase space using the energy landscape visualization method. (a) Each conformation displayed by a point in the effective phase space. (b) Contour plots showing the density of states of the wild type (WT) and phosphorylated PAGE4. Each free energy valley is characterized by specific conformations that entail particular binding affinities. For WT-PAGE4, through a fly-casting mechanism, the C-terminal region is extended, facilitating its interaction with partner protiens. In the case of HIPK1-PAGE4, the lower free energy of the compact state decreases the affinity for c-Jun, while the extended conformations of CLK2-PAGE4 due to hyperphosporylation inhibit this interaction.
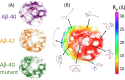
Conformational phase space of the simulated amyloid-β (Ab) structures. (a) Distinct Ab ensembles present in the projection with the Ab-40 (purple), Ab-42 (beige), and the Ab-40 mutants (green). (b) Each point represents a conformation, which is colored as function of radius of gyration. Typical conformation examples of each region are displayed around the projection.
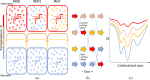
Schematic diagram of different parallel tempering simulations. (a) In parallel tempering simulations, a series of low and high temperature replicas are simulated. The replicas in the conventional REMD differ by increasing bath temperatures across the ladder. Thus, the probability of accepting the exchange between adjacent replicas depends on the difference in the complete Hamiltonian of the system, including solute–solute, solute–solvent, and solvent–solvent contributions, which results in poor scaling in large systems. REST2 scales the energy function in a particle-wise manner, such that the solute is effectively heated up while keeping the solvent cold. Thus, the exchange acceptance probability depends on the energy difference in solute–solute interactions mainly and solute–solvent interactions subtly. The imbalance between hot solute and cold solvent causes entropic trap. REHT optimally heats the solute as well as the surrounding solvent by associating the replicas to different bath temperatures in addition to scaling down the potential function. Note that the base replica in all the cases is unbiased and has the same forcefield parameters and temperature conditions. (b) Stochastic swapping of replicas at regular intervals facilitates the equilibrium sampling at the base replica. (c) Schematic of energy landscape illustrating accessibility of broader conformational space facilitated by the high temperature replica.
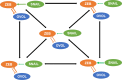
Dynamic protein interaction networks. A “static” network consisting of IDPs (center) can be altered in terms of relative strengths of interactions across the nodes, in the presence of conformational noise. Orange hammer shows inhibition; green arrows show activation. Thicker lines show stronger activation or inhibition than weaker ones. These “dynamic” networks can interchange among themselves as well due to changes in conformational structure of IDPs/IDPRs involved.
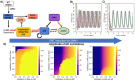
Coupled dynamics of EMT and PAGE4 circuits containing IDPs. (a) Coupled network of EMT and PAGE4 circuit. Solid red hammer heads correspond to transcriptional inhibition, and dotted red hammer heads correspond to post transcriptional inhibition due to micro-RNA interaction. Solid black arrows correspond to transcriptional activation, and dotted arrows stand for phosphorylation. (b) and (c) Dynamics of AR: AR levels over time for two different values of interaction strength with ZEB. (d) Induction of EMT via SNAI1 leads to oscillations converting to bistability. Phase plot between interaction strengths of AR and Zeb, as EMT is induced via SNAI1, Zeb inhibits AR more strongly and leads to oscillations converting to bistability. [Adapted from Singh et al., Entropy (Basel) 23(3), 288 (2021). Copyright 2021 MDPI].
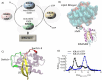
The structure/function cycle of KRAS. (a) Activation/deactivation cycle of KRAS GTPase. GDP/GTP exchange in this cycle is mediated by two other proteins: guanine nucleotide-exchange factors (GEFs) and GTPase activating proteins (GAPs). While GEFs catalyze the exchange from GDP to GTP, GAPs enhance the rate of exchange from GTP to GDP. (b) NMR-driven structure of KRAS4B-GTP on a lipid bilayer (pdb id:6W4E) (Lee et al., 2020). The positively charged intrinsically disordered hypervariable region (HVR) is shown in gray to highlight its mode of association with the lipid membrane. (c) Three-dimensional structure of inactive GDP-bound human KRAS highlighting the dynamic switch regions: Switch I (green) and Switch II (red) (pdb id: 4OBE) (Hunter et al., 2014). These two switch regions are connected via two parallel β strands: β2 and β3. (d) The switch dynamics and their correlation with β2-β3 fluctuation are compared in GDP and GTP-bound states. The dynamics are assessed by quantifying the distance between two residues R41 (located in β2) and D54 (located in β3). The distance distribution indicates enhanced conformation fluctuation of the switches in the GDP-bound state. [Adapted from Vatansever et al., Sci. Rep. 6, 37012 (2016). Copyright 2016 Author(s), licensed under a Creative Commons Attribution 4.0 License.]
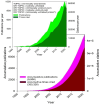
An explosion in the protein intrinsic disorder literature. The plots represent the time-courses of the increase in the number of publications dealing with the intrinsic disorder and the number of papers citing those publications (inset). Plot shows total publications per year and accumulative number of publications. Inset shows the data for the sum of times cited per year and accumulative sum of times cited. Data for these plots were retrieved from Web of Science on November 17, 2021 using the following search criteria: TOPIC: (intrinsically disordered) OR TOPIC: (natively unfolded) OR TOPIC: (intrinsically unstructured) OR TOPIC: (natively unstructured) OR TOPIC: (intrinsically unfolded protein).
Similar articles
-
Protein conformational dynamics and phenotypic switching.
Kulkarni P, Achuthan S, Bhattacharya S, Jolly MK, Kotnala S, Leite VBP, Mohanty A, Orban J, Roy S, Rangarajan G, Salgia R. Kulkarni P, et al. Biophys Rev. 2021 Nov 27;13(6):1127-1138. doi: 10.1007/s12551-021-00858-x. eCollection 2021 Dec. Biophys Rev. 2021. PMID: 35059032 Free PMC article. Review.
-
Recent Advances in Computational Protocols Addressing Intrinsically Disordered Proteins.
Bhattacharya S, Lin X. Bhattacharya S, et al. Biomolecules. 2019 Apr 11;9(4):146. doi: 10.3390/biom9040146. Biomolecules. 2019. PMID: 30979035 Free PMC article. Review.
-
Intrinsically disordered proteins and conformational noise: implications in cancer.
Mahmoudabadi G, Rajagopalan K, Getzenberg RH, Hannenhalli S, Rangarajan G, Kulkarni P. Mahmoudabadi G, et al. Cell Cycle. 2013 Jan 1;12(1):26-31. doi: 10.4161/cc.23178. Epub 2012 Dec 19. Cell Cycle. 2013. PMID: 23255110 Free PMC article.
-
Zhu JJ, Zhang NJ, Wei T, Chen HF. Zhu JJ, et al. Int J Mol Sci. 2023 Apr 7;24(8):6896. doi: 10.3390/ijms24086896. Int J Mol Sci. 2023. PMID: 37108059 Free PMC article.
-
Backbone conformational preferences of an intrinsically disordered protein in solution.
Espinoza-Fonseca LM, Ilizaliturri-Flores I, Correa-Basurto J. Espinoza-Fonseca LM, et al. Mol Biosyst. 2012 Jun;8(6):1798-805. doi: 10.1039/c2mb00004k. Epub 2012 Apr 13. Mol Biosyst. 2012. PMID: 22506277
Cited by
-
Appadurai R, Koneru JK, Bonomi M, Robustelli P, Srivastava A. Appadurai R, et al. J Chem Theory Comput. 2023 Jul 25;19(14):4711-4727. doi: 10.1021/acs.jctc.3c00224. Epub 2023 Jun 20. J Chem Theory Comput. 2023. PMID: 37338049 Free PMC article.
-
Analysis of proteins in the light of mutations.
Vila JA. Vila JA. Eur Biophys J. 2024 Aug;53(5-6):255-265. doi: 10.1007/s00249-024-01714-y. Epub 2024 Jul 2. Eur Biophys J. 2024. PMID: 38955858 Review.
-
Gupta MN, Uversky VN. Gupta MN, et al. Protein Sci. 2024 Apr;33(4):e4968. doi: 10.1002/pro.4968. Protein Sci. 2024. PMID: 38532700 Review.
-
Functional Resilience of Mutually Repressing Motifs Embedded in Larger Networks.
Harlapur P, Duddu AS, Hari K, Kulkarni P, Jolly MK. Harlapur P, et al. Biomolecules. 2022 Dec 9;12(12):1842. doi: 10.3390/biom12121842. Biomolecules. 2022. PMID: 36551270 Free PMC article.
-
Addressing Drug Resistance in Cancer: A Team Medicine Approach.
Kulkarni P, Mohanty A, Bhattacharya S, Singhal S, Guo L, Ramisetty S, Mirzapoiazova T, Mambetsariev B, Mittan S, Malhotra J, Gupta N, Kim P, Babikian R, Rajurkar S, Subbiah S, Tan T, Nguyen D, Merla A, Kollimuttathuillam SV, Phillips T, Baik P, Tan B, Vashi P, Shrestha S, Leach B, Garg R, Rich PL, Stewart FM, Pisick E, Salgia R. Kulkarni P, et al. J Clin Med. 2022 Sep 27;11(19):5701. doi: 10.3390/jcm11195701. J Clin Med. 2022. PMID: 36233569 Free PMC article.
References
-
- Adamski, W. , Salvi, N. , Maurin, D. , Magnat, J. , Milles, S. , Jensen, M. R. , Abyzov, A. , Moreau, C. J. , and Blackledge, M. , “ A unified description of intrinsically disordered protein dynamics under physiological conditions using NMR spectroscopy,” J. Am. Chem. Soc. 141(44), 17817–17829 (2019).10.1021/jacs.9b09002 - DOI - PubMed
Publication types
LinkOut - more resources
Full Text Sources