Zinc Nitrate Hexahydrate Pseudobinary Eutectics for Near-Room-Temperature Thermal Energy Storage - PubMed
- ️Sun Jan 01 2023
Review
Zinc Nitrate Hexahydrate Pseudobinary Eutectics for Near-Room-Temperature Thermal Energy Storage
Sophia Ahmed et al. ACS Appl Eng Mater. 2023.
Abstract
Stoichiometric salt hydrates can be inexpensive and provide higher volumetric energy density relative to other near-room-temperature phase change materials (PCMs), but few salt hydrates exhibit congruent melting behavior between 0 and 30 °C. Eutectic salt hydrates offer a strategy to design bespoke PCMs with tailored application-specific eutectic melting temperatures. However, the general solidification behavior and stability of eutectic salt hydrate systems remain unclear, as metastable solidification in eutectic salt hydrates may introduce opportunities for phase segregation. Here, we present a new family of low-cost zinc-nitrate-hexahydrate-based eutectics: Zn(NO3)2·6(H2O)-NaNO3 (Teu = 32.7 ± 0.3 °C; ΔHeu = 151 ± 6 J·g-1), Zn(NO3)2·6(H2O)-KNO3 (Teu = 22.1 ± 0.3 °C; ΔHeu = 140 ± 6 J·g-1), Zn(NO3)2·6(H2O)-NH4NO3 (Teu = 11.2 ± 0.3 °C; ΔHeu = 137 ± 5 J·g-1). While the tendency to undercool varies greatly between different eutectics in the family, the geologic mineral talc has been identified as an active and stable phase that dramatically reduces undercooling in Zn(NO3)2·6(H2O) and all related eutectics. Zn(NO3)2·6(H2O) and its related eutectics have shown stability for over a hundred thermal cycles in mL scale volumes, suggesting that they are capable of serving as robust and stable media for near-room-temperature thermal energy storage applications in buildings.
© 2023 American Chemical Society.
Conflict of interest statement
The authors declare no competing financial interest.
Figures
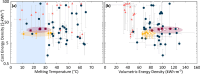
Cost-energy density as a function of (a) melting temperature of the PCM and (b) volumetric energy density of the PCM with reference data taken from Hirschey et al. and Ahmed et al., Figure adapted with permission from ref (7). Copyright 2021, retained by authors. Solid shapes represent salt hydrates in the liquid phase, and outlined represent the solid phase. Yellow diamonds are Zn(NO3)2·6H2O eutectics (reported here), maroon squares are LiNO3·3(H2O) eutectics, pink triangles are paraffin, and blue circles are other salt hydrates. The blue shaded section in (a) indicates the desired temperature range for TES media in buildings.
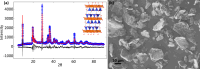
(a) The observed powder diffraction pattern of talc in blue, with the calculated spectra illustrated in red, and a difference curve in black. A supercell of the resultant crystal structure of talc in the top right corner, showing the layering of tetrahedral-octahedral-tetrahedral sites observed in talc, resulting in a pseudohexagonal in-plane structure. (b) SEM image of talc taken using secondary electrons at 10 keV. The image shows the flaky nature of talc.
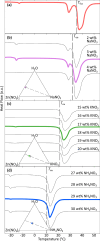
DSC curve of ZNH (a) as well as DSC curves for composition arrays of tested pseudobinary ZNH eutectics, ZNH-NaNO3 (b, purple), ZNH-KNO3 (c, green), and ZNH-NH4NO3 (d, blue), as measured at heating and cooling rates of 10 °C·min–1 to determine the eutectic composition to within 1 wt % (highlighted in bold and in color). Dashed lines refer to the reported Tfus or Teu collected from the Q2000 DSC. Eutectic compositions were selected for having a narrow peak width, indicative of minima in the liquidus temperature (associated with the eutectic point). Mixtures with higher concentrations of anhydrous nitrate are above the solubility limit at room temperature. A ternary phase diagram is shown on each plot with a dot indicative of eutectic composition, and a tick mark indicative of solublity limit.
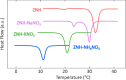
Resultant DSC curves of ZNH and related eutectics taken at 1 °C·min–1 on Microcalvet DSC.
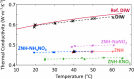
Thermal conductivity of liquid ZNH and related eutectics, compared to water, measured on both heating and cooling. Deionized water (DIW) is provided for comparison as well as reference values from NIST. The black triangle at 40 °C is a previously reported thermal conductivity for ZNH from Lane.
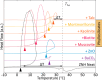
Various NPs were tested alongside neat ZNH to determine the effect on undercooling. 1 wt % quantities of NP were added to a DSC pan with ZNH.
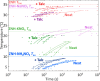
Isothermal crystallization times at various temperatures for ZNH (red) and eutectics containing NaNO3 (purple), KNO3 (green), and NH4NO3 (blue) in the presence and absence of the talc nucleation particles. Darker shades of the two colors presented at a temperature represent samples that contain 2 wt % of talc. Curves represent isothermal nucleation times for 25, 50, and 75% probability of nucleation, calculated as described in the text.
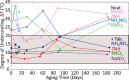
Samples were aged for short periods and periodically sampled in hermetically sealed DSC pans initially (dashed line), and were then extracted from vials and measured (solid line). Neat systems (light shades) were compared with talc-inclusive systems (dark shades).
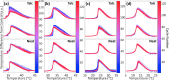
Calculated difference between the cell thermocouple reading and the control thermocouple in an unhoused cell are plotted against temperature for (a) ZNH, (b) ZNH-NaNO3, (c) ZNH-KNO3, and (d) ZNH-NH4NO3. The top two curves of each plot are talc-inclusive samples, and the bottom two are neat samples.
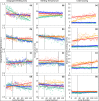
Three sets of graphs for: (left) normalized integrated area of the melting curves, (middle) melting temperature, and (right) degrees of undercooling for (a) ZNH, (b) ZNH-NaNO3, (c) ZNH-KNO3, and (d) ZNH-NH4NO3, over many cycles. Red and orange points indicate neat samples A and B respectively for each system, and blue and green indicate talc samples A and B for each system. The vertical line indicates a pause between sets of cycles in all systems but ZNH where it did not have one.
Similar articles
-
Printing Composites with Salt Hydrate Phase Change Materials for Thermal Energy Storage.
Lak SN, Hsieh CM, AlMahbobi L, Wang Y, Chakraborty A, Yu C, Pentzer EB. Lak SN, et al. ACS Appl Eng Mater. 2023 Aug 4;1(8):2279-2287. doi: 10.1021/acsaenm.3c00324. eCollection 2023 Aug 25. ACS Appl Eng Mater. 2023. PMID: 38356854 Free PMC article.
-
Thermal Storage of Nitrate Salts as Phase Change Materials (PCMs).
Orozco MA, Acurio K, Vásquez-Aza F, Martínez-Gómez J, Chico-Proano A. Orozco MA, et al. Materials (Basel). 2021 Nov 26;14(23):7223. doi: 10.3390/ma14237223. Materials (Basel). 2021. PMID: 34885375 Free PMC article.
-
Highly Stable Energy Capsules with Nano-SiO2 Pickering Shell for Thermal Energy Storage and Release.
Graham M, Smith J, Bilton M, Shchukina E, Novikov AA, Vinokurov V, Shchukin DG. Graham M, et al. ACS Nano. 2020 Jul 28;14(7):8894-8901. doi: 10.1021/acsnano.0c03706. Epub 2020 Jun 17. ACS Nano. 2020. PMID: 32539347 Free PMC article.
-
Rajagopalan KK, Haney S, Shamberger PJ, Sukhishvili SA. Rajagopalan KK, et al. ACS Appl Eng Mater. 2023 Dec 8;2(3):553-562. doi: 10.1021/acsaenm.3c00522. eCollection 2024 Mar 22. ACS Appl Eng Mater. 2023. PMID: 38544947 Free PMC article. Review.
-
Eutectics: formation, properties, and applications.
Yu D, Xue Z, Mu T. Yu D, et al. Chem Soc Rev. 2021 Aug 2;50(15):8596-8638. doi: 10.1039/d1cs00404b. Chem Soc Rev. 2021. PMID: 34138999 Review.
Cited by
-
Mutability of Nucleation Particles in Reactive Salt Hydrate Phase Change Materials.
Ibbotson D, Ahmed S, Shamberger PJ. Ibbotson D, et al. J Phys Chem C Nanomater Interfaces. 2024 Oct 7;128(41):17282-17290. doi: 10.1021/acs.jpcc.4c03913. eCollection 2024 Oct 17. J Phys Chem C Nanomater Interfaces. 2024. PMID: 39439884 Free PMC article.
References
-
- Du K.; Calautit J.; Wang Z.; Wu Y.; Liu H. A Review of the Applications of Phase Change Materials in Cooling, Heating and Power Generation in Different Temperature Ranges. Appl. Energy 2018, 220, 242–273. 10.1016/j.apenergy.2018.03.005. - DOI
-
- Henry A.; Prasher R.; Majumdar A. Five Thermal Energy Grand Challenges for Decarbonization. Nat. Energy 2020, 5 (9), 635–637. 10.1038/s41560-020-0675-9. - DOI
-
- Lane G. A. Low Temperature Heat Storage With Phase Change Materials. Int. J. Ambient Energy 1980, 1 (3), 155–168. 10.1080/01430750.1980.9675731. - DOI
-
- Zeng D.; Voigt W. Phase Diagram Calculation of Molten Salt Hydrates Using the Modified BET Equation. CALPHAD 2003, 27, 243–251. 10.1016/j.calphad.2003.09.004. - DOI
-
- Schmit H.; Rathgeber C.; Hennemann P.; Hiebler S. Three–Step Method To Determine the Eutectic Composition of Binary and Ternary Mixtures. J. Therm. Anal. Calorim. 2014, 117 (2), 595–602. 10.1007/s10973-014-3783-6. - DOI
Publication types
LinkOut - more resources
Full Text Sources