Condensin-mediated restriction of retrotransposable elements facilitates brain development in Drosophila melanogaster - PubMed
- ️Mon Jan 01 2024
Condensin-mediated restriction of retrotransposable elements facilitates brain development in Drosophila melanogaster
Bert I Crawford et al. Nat Commun. 2024.
Erratum in
-
Crawford BI, Talley MJ, Russman J, Riddle J, Torres S, Williams T, Longworth MS. Crawford BI, et al. Nat Commun. 2025 Jan 22;16(1):933. doi: 10.1038/s41467-025-56152-x. Nat Commun. 2025. PMID: 39843423 Free PMC article. No abstract available.
Abstract
Neural stem and progenitor cell (NSPC) maintenance is essential for ensuring that organisms are born with proper brain volumes and head sizes. Microcephaly is a disorder in which babies are born with significantly smaller head sizes and cortical volumes. Mutations in subunits of the DNA organizing complex condensin have been identified in microcephaly patients. However, the molecular mechanisms by which condensin insufficiency causes microcephaly remain elusive. We previously identified conserved roles for condensins in repression of retrotransposable elements (RTEs). Here, we show that condensin subunit knockdown in NSPCs of the Drosophila larval central brain increases RTE expression and mobility which causes cell death, and significantly decreases adult head sizes and brain volumes. These findings suggest that unrestricted RTE expression and activity may lead to improper brain development in condensin insufficient organisms, and lay the foundation for future exploration of causative roles for RTEs in other microcephaly models.
© 2024. The Author(s).
Conflict of interest statement
The authors declare no competing interests.
Figures
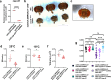
a qRT-PCR analyses of cap-d3 transcripts were performed on cDNA generated from first instar larvae expressing control UAS-GFP dsRNA (pink) or UAS-cap-d3 dsRNA (red) under the control of armGAL4. Transcripts were normalized to the housekeeping gene, rp49. The chart shown includes technical replicates and is representative of two independent experiments. P values were determined by performing a two-tailed, unpaired t-test. b Adult female fly heads were dissected from flies expressing control UAS-GFP dsRNA (top row) or UAS-cap-d3 dsRNA (bottom row) under the control of eyGAL4, GMRGAL4. c–e The distance between two machrochaetes positioned between the eyes of adult flies was measured as a proxy for measuring adult head size. The region measured is shown in (c). Adult, female fly head sizes were measured on flies expressing control UAS-GFP dsRNA (pink) or UAS-cap-d3 dsRNA (red) driven by eyGAL4, GMRGAL4 at 25 °C (d) or at 18 °C (e). Charts shown in (d) and (e) are representative of two independent experiments; each data point represents a single fly. f Adult brain volumes were measured in female flies (1–3 days post-eclosure) expressing control UAS-GFP dsRNA (pink) or UAS-cap-d3 dsRNA (red) driven by eyGAL4, GMRGAL4 at 25 °C. The charts shown are representative of two independent experiments; each data point represents a single brain. g Adult head sizes were measured in female flies expressing different combinations of control UAS-GFP dsRNA or UAS-cap-d3 dsRNA and UAS-GFP or UAS-GFP-cap-d3 under the control of eyGAL4, GMRGAL4. The results shown are the combined results of two independent experiments; each data point represents a single fly. For experiments in (d–g), P values were determined by performing two-tailed Mann–Whitney analyses. For a, p ≤ 0.0001. For d, p = 0.0001. For e, p = 0.0121. For f, p = 0.0010. For g, **p = 0.0060, ***p = 0.0001, ****p ≤ 0.0001. NS not significant. For all experiments, error bars indicate standard deviations from the mean.
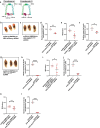
a Diagram of the proteins that comprise the condensin I and condensin II complexes in Drosophila melanogaster. b, c, g) Female pupae expressing (b) UAS-GFP dsRNA, (c) smc2 dsRNA, or (g) cap-d2 dsRNA under the control of eyGAL4, GMRGAL4 were dissected from pupal cases and imaged. e, i, k Adult, female fly head sizes were measured from flies expressing control (UAS-GFP or UAS-mCherry) dsRNA or dsRNA targeting (e) smc2, (i) cap-d2, and (k) cap-h under the control of eyGAL4, GMRGAL4. The charts shown are representative of two independent experiments; each data point represents a single fly. P values were determined by performing two-tailed Mann–Whitney analyses. For (e), p = 0.0010. For (i), p = 0.0376. For (k), p = 0.0067. d, f, h, j, l qRT-PCR analyses of d, f smc2, h, j cap-d2, and l cap-h transcript levels were performed on cDNA generated from first instar larvae expressing control (UAS-GFP or UAS-mCherry) dsRNA or dsRNAs targeting the respective condensin subunit mRNA under the control of armGAL4. Transcripts were normalized to the housekeeping gene, rp49. The charts shown include technical replicates of samples collected from four independent experiments (~200 larvae per experiment, combined into one sample per genotype). P values were determined by performing two-tailed, unpaired t-tests. For (d), p = 0.0021. For (f), p = 0.0421. For (h), p ≤ 0.0001. For all experiments, error bars indicate standard deviations from the mean.
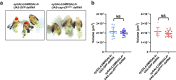
a Larvae expressing control UAS-GFP dsRNA or UAS-cap-d3 dsRNA, under the control of eyGAL4, GMRGAL4 were allowed to pupate for 72–96 h, at which time males were dissected and imaged. b The volumes of brains dissected from third instar larvae expressing control UAS-GFP dsRNA or UAS-cap-d3 dsRNA, under the control of eyGAL4, GMRGAL4 were measured in males (left) and females (right). Results shown include larvae harvested from two independent experiments; each data point represents a single brain. P values were determined by performing two-tailed Mann–Whitney analyses. NS not significant. Error bars indicate standard deviations from the mean.
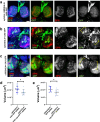
a–c Third instar larval brains were dissected from male larvae expressing UAS-GFP under the control of a eyGAL4, GMRGAL4, b optixGAL4, or c c253GAL4, immunostained with antibodies to detect GFP (green) and stem cell marker, Dpn (red), and imaged using confocal microscopy. Maximum projections of z-stacks are shown. Nuclei are stained with DAPI (blue). All images were taken with 40x magnification. All images shown are representative of at least five biological replicates. Scale bar = 50 µm. d, e Adult brain volumes were measured in male flies expressing control UAS-GFP dsRNA or UAS-cap-d3 dsRNA driven by d optixGAL4 or e c253GAL4 at 25 °C; each data point represents a single brain. P values were determined by performing two-tailed Mann–Whitney analyses. For (d), p = 0.0156. For (e), p = 0.0278. Error bars indicate standard deviations from the mean. Results shown include adults harvested from two independent experiments.
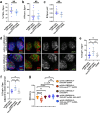
a Third instar larval brains were dissected from male larvae expressing control UAS-GFP dsRNA or UAS-cap-d3 dsRNA, under the control of eyGAL4, GMRGAL4, immunostained with antibodies to detect stem cell marker, Dpn, and mitotic cell marker, PH3, and imaged using confocal microscopy. The percentage of cells that stained positive for both Dpn and PH3 were quantified. b Immunostaining for Dpn and Pros, a marker of differentiation, was performed in male third instar larval brains. The number of cells that stained positive for both Dpn and Pros were quantified. c The number of Dpn-positive cells per square micrometer of larval central brain tissue in larvae expressing cap-d3 dsRNA or control, gfp dsRNA was quantified. For a–c, results shown include larvae harvested from two independent experiments; each data point represents a single brain. P values were determined by performing two-tailed Mann–Whitney analyses. NS not significant. d Immunostaining to detect Dpn and the cell death marker, Dcp1 was performed in male third instar larval brains expressing control UAS-GFP dsRNA (dark blue) or UAS-cap-d3 dsRNA (light blue), under the control of eyGAL4, GMRGAL4. Images were taken with a confocal microscope. Maximum projections of z-stacks are shown. Nuclei are stained with DAPI (blue). All images were taken with 40x magnification. Scale bar = 50 µm. e The percentage of cells that stained positive for both Dpn and Dcp1 in (d) were quantified. Additionally, larval brains expressing eyGAL4 and GMRGAL4 (gray; no RNAi control) were imaged and quantified. Each data point represents a single brain. f Dcp1+, Dpn− cells located adjacent to Dpn-positive cells in male third instar larval brains (experiments described in d, e) were quantified. Each data point represents a single brain. g Adult female head sizes were measured in female flies expressing different combinations of control UAS-GFP dsRNA or UAS-cap-d3 dsRNA and the Drosophila inhibitor of apoptosis, UAS-diap1, under the control of eyGAL4, GMRGAL4. For e–g, results shown include larvae harvested from two independent experiments; each data point represents a single brain (e, f) or a single fly (g). P values were determined by performing two-tailed Mann–Whitney analyses. For (e), *p = 0.0212 and *#p = 0.0411. For (f), *p = 0.0013 and *#p = 0.0024. For (g), *p = 0.0422, ***p = 0.0004, ****p ≤ 0.0001, ****#p ≤ 0.0001, NS not significant. For all experiments, error bars indicate standard deviations from the mean.
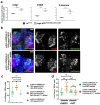
a qRT-PCR analyses of RTE (mdg1, mdg4, and X-element) transcripts were performed with cDNA generated from third instar larval brains dissected from w1118 controls or cap-d3 transheterozygous mutant larvae (150 brains per genotype; mixed sexes). Transcripts were normalized to the housekeeping gene, rp49. Results show the averages of three independent biological replicates. P values were determined by performing two-tailed, unpaired t-tests. For mdg1, **p = 0.0054, for mdg4, **p = 0.0015, and for x-element, **p = 0.0067. b Third instar larval brains were dissected from male larvae expressing UAS-gypsyCLEVR and UAS-GFP dsRNA or UAS-cap-d3 dsRNA under the control of eyGAL4, GMRGAL4, immunostained with antibodies to mCherry, a reporter of gypsy retrotransposition (pseudocolored green), and stem cell marker, Dpn (red), and imaged using confocal microscopy. Maximum projections of z-stacks are shown. Nuclei are stained with DAPI (blue). All images were taken with 40x magnification. Scale bar = 50 µm. c The percentage of cells staining positive for both mCherry and Dpn were quantified from experiments described in (b). Male larval brains expressing eyGAL4 and GMRGAL4-driven UAS-gypsyCLEVR were also analyzed as “no RNAi” controls. Results shown include larvae harvested from two independent experiments; each data point represents a single brain. d Third instar larval brains were dissected from male larvae expressing UAS-gypsyCLEVR and control UAS-GFP dsRNA (green circles) or UAS-cap-d3 dsRNA (orange squares), under the control of eyGAL4, GMRGAL4, immunostained with antibodies to detect mCherry and Dpn, and imaged using confocal microscopy. The percentage of cells that stained positive for both Dpn and Dcp1 were quantified. Flies were developed on food containing DMSO as a control (closed circles and squares), or on food containing 5 µM AZT (open circles and squares). Results shown include larvae harvested from two independent experiments; each data point represents a single brain. For experiments in (c, d), P values were determined by performing two-tailed Mann–Whitney analyses. For (c), **p = 0.0029, ****p ≤ 0.0001, NS not significant. For (d), **p = 0.0037, **#p = 0.0039, NS not significant. Error bars indicate standard deviations from the mean.
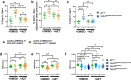
a Third instar larval brains were dissected from female larvae expressing control UAS-GFP dsRNA (green circles) or UAS-cap-d3 dsRNA (orange squares), under the control of eyGAL4, GMRGAL4, immunostained with antibodies to detect stem cell marker, Dpn, and cell death marker, Dcp1, and imaged using confocal microscopy. The percentage of cells that stained positive for both Dpn and Dcp1 were quantified. Larvae were developed on food containing DMSO as a control (closed circles and squares), or on food containing 5 µM AZT (open circles and squares). b Dcp1+, Dpn− cells located adjacent to Dpn-positive cells in female third instar larval brains (experiments described in (a)) were quantified. Results shown in (a, b) include larvae harvested from two independent experiments; each data point represents a single brain. c Experiments described in (a) were performed on brains dissected from cap-d3 transheterozygous mutant (blue squares) male larvae or wild-type (green circles) male larvae. Results shown include larvae harvested from two independent experiments; each data point represents a single brain. d, e Adult female fly head sizes were measured on flies expressing control UAS-GFP dsRNA (green circles) or UAS-cap-d3 dsRNA (orange squares) driven by eyGAL4, GMRGAL4 at 25 °C. Flies were developed on food containing DMSO as a control (closed circles and squares), or on food containing (d) 5 µM AZT or (e) 5 µM d4T (open circles and squares). f Adult male head sizes were measured on wild-type flies (green circles), on cap-d3 transheterozygotes (sky blue squares) or cap-d3 heterozygotes (light blue and dark blue squares). Flies were developed on food containing DMSO as a control (closed circles and squares), or on food containing 5 µM AZT (open circles and squares). Results shown in (d–f) include adults harvested from two independent experiments; each data point represents a single fly. P values were determined by performing two-tailed Mann–Whitney analyses. For (a), ****p ≤ 0.0001 and ****# p ≤ 0.0001. For (b), *p = 0.0434, **p = 0.0079, NS not significant. For (c), **p = 0.0013, ***p = 0.0007, NS not significant. For (d), *p = 0.0208, *#p = 0.0336, **p = 0.0014. For (e), *p = 0.038, **p = 0.0015, **#p = 0.0084, NS not significant. For (f), *p = 0.0181, **p = 0.0018, ****p ≤ 0.0001, ****#p ≤ 0.0001, NS not significant. For all experiments, error bars indicate standard deviations from the mean.
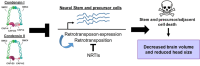
Condensin I and II restrict RTE expression and retrotransposition in neuroblasts and intermediate neural precursor cells in the developing larval brain. This, in turn, restricts cell death of stem and precursor cells, as well as cells adjacent to stem/precursor cells, thereby allowing the brain to achieve proper volume by the late pupal stages.
Similar articles
-
Bozler J, Nguyen HQ, Rogers GC, Bosco G. Bozler J, et al. G3 (Bethesda). 2014 Dec 30;5(3):341-52. doi: 10.1534/g3.114.015685. G3 (Bethesda). 2014. PMID: 25552604 Free PMC article.
-
Far Posterior Approach for Rib Fracture Fixation: Surgical Technique and Tips.
Manes TJ, DeGenova DT, Taylor BC, Patel JN. Manes TJ, et al. JBJS Essent Surg Tech. 2024 Dec 6;14(4):e23.00094. doi: 10.2106/JBJS.ST.23.00094. eCollection 2024 Oct-Dec. JBJS Essent Surg Tech. 2024. PMID: 39650795 Free PMC article.
-
Depressing time: Waiting, melancholia, and the psychoanalytic practice of care.
Salisbury L, Baraitser L. Salisbury L, et al. In: Kirtsoglou E, Simpson B, editors. The Time of Anthropology: Studies of Contemporary Chronopolitics. Abingdon: Routledge; 2020. Chapter 5. In: Kirtsoglou E, Simpson B, editors. The Time of Anthropology: Studies of Contemporary Chronopolitics. Abingdon: Routledge; 2020. Chapter 5. PMID: 36137063 Free Books & Documents. Review.
-
Radiation Effects On The Fetus.
Kumar R, De Jesus O. Kumar R, et al. 2023 Aug 23. In: StatPearls [Internet]. Treasure Island (FL): StatPearls Publishing; 2025 Jan–. 2023 Aug 23. In: StatPearls [Internet]. Treasure Island (FL): StatPearls Publishing; 2025 Jan–. PMID: 33232028 Free Books & Documents.
-
Quinonez SC, Lee KN. Quinonez SC, et al. 2004 Jul 7 [updated 2022 Aug 18]. In: Adam MP, Feldman J, Mirzaa GM, Pagon RA, Wallace SE, Amemiya A, editors. GeneReviews® [Internet]. Seattle (WA): University of Washington, Seattle; 1993–2025. 2004 Jul 7 [updated 2022 Aug 18]. In: Adam MP, Feldman J, Mirzaa GM, Pagon RA, Wallace SE, Amemiya A, editors. GeneReviews® [Internet]. Seattle (WA): University of Washington, Seattle; 1993–2025. PMID: 20301631 Free Books & Documents. Review.
Cited by
-
Retrotransposons in embryogenesis and neurodevelopment.
Talley MJ, Longworth MS. Talley MJ, et al. Biochem Soc Trans. 2024 Jun 26;52(3):1159-1171. doi: 10.1042/BST20230757. Biochem Soc Trans. 2024. PMID: 38716891 Free PMC article. Review.
References
-
- Homem, C. C. & Knoblich, J. A. Drosophila neuroblasts: a model for stem cell biology. Development139, 4297–4310 (2012). - PubMed
-
- Hobbs, K. et al. A retrospective fetal ultrasound study of brain size in autism. Biol. Psychiatry62, 1048–1055 (2007). - PubMed
-
- Sacco, R., Gabriele, S. & Persico, A. M. Head circumference and brain size in autism spectrum disorder: a systematic review and meta-analysis. Psychiatry Res.234, 239–251 (2015). - PubMed
MeSH terms
Substances
LinkOut - more resources
Full Text Sources
Molecular Biology Databases