2D Halide Perovskites for High-Performance Resistive Switching Memory and Artificial Synapse Applications - PubMed
Review
2D Halide Perovskites for High-Performance Resistive Switching Memory and Artificial Synapse Applications
Bixin Li et al. Adv Sci (Weinh). 2024 Jun.
Abstract
Metal halide perovskites (MHPs) are considered as promising candidates in the application of nonvolatile high-density, low-cost resistive switching (RS) memories and artificial synapses, resulting from their excellent electronic and optoelectronic properties including large light absorption coefficient, fast ion migration, long carrier diffusion length, low trap density, high defect tolerance. Among MHPs, 2D halide perovskites have exotic layered structure and great environment stability as compared with 3D counterparts. Herein, recent advances of 2D MHPs for the RS memories and artificial synapses realms are comprehensively summarized and discussed, as well as the layered structure properties and the related physical mechanisms are presented. Furthermore, the current issues and developing roadmap for the next-generation 2D MHPs RS memories and artificial synapse are elucidated.
Keywords: 2D halide perovskite; Ruddlesden–Popper; artificial synapses; resistive switching memory.
© 2024 The Authors. Advanced Science published by Wiley‐VCH GmbH.
Conflict of interest statement
The authors declare no conflict of interest.
Figures
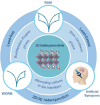
Overview of 2D MHPs memristors.
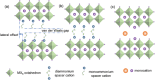
Illustration of 2D MHPs phases: a) RP phase; b) DJ phase; c) ACI phase.
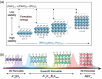
a) The evolution of dimensionality and formation energy as a function of n. Reprinted with permission.[ 55 ] Copyright 2016, American Chemical Society. b) Schematic illustration of perovskites bandgap and dimensionality engineering relationship. Reprinted with permission.[ 54 ] Copyright 2018, Wiley‐VCH.
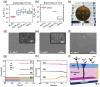
a) The electric field (E), and b) the ON/OFF ratio (under 0.02 V) for the different MHPs from BA2PbI4 to MAPbI3. c) 4 inch‐wafer BA2PbI4/Ag deposited on the (1) to (5). Reprinted with permission.[ 32 ] Copyright 2017, The Royal Society of Chemistry. d–f) SEM images of the BA2PbBr4 film at 100, 150, and 200 °C, respectively. Reprinted with permission.[ 66 ] Copyright 2019, American Chemical Society. g) Four different current states at different voltage. h) Retention stability of “11”, “10”, “01”. Reprinted with permission.[ 67 ] Copyright 2019, American Chemical Society. i) Device structure of the switching device composed of BA2PbBr4 and the Ag‐doped ZnO (AZO) layer. Reprinted with permission.[ 68 ] Copyright 2021, American Chemical Society.
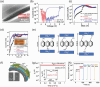
a) SEM image of an PEA2PbBr4 single crystal. b) Typical electrical curves for set and reset process of PEA2PbBr4 single crystal device. Reprinted with permission.[ 70 ] Copyright 2017, American Chemical Society. c) I−V curves under three different scans: from 0 to 6 V with an initial OFF state and from 6 to 0 V and from 0 to 6 V with an initial ON state for ITO/PEDOT:PSS/perovskite/Bphen/Ag device. d) I−V curves under three different scans. The inset shows the device structure of ITO/MAPbBr3/MAPbBr3/ITO. Reprinted with permission.[ 71 ] Copyright 2020, American Chemical Society. e) Schematic illustration of the interlayer structure of (An)2PbI4, (BzA)2PbI4, and (PEA)2PbI4. Reprinted with permission.[ 72 ] Copyright 2019, The Royal Society of Chemistry. f) Device structure and SEM image of flexible memory. g) The storage stability of the memory behavior (Plight = 6.8 mW cm−2). h) Multilevel memory property of the optoelectronic memory. Reprinted with permission.[ 73 ] Copyright 2022, American Chemical Society.
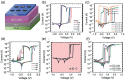
a) Schematic illustration of Ag/PMMA/(BzA)2CuBr4/Pt/Ti/SiO2/Si. b) I–V characteristics. c) I–V characteristics under different compliance currents. Reprinted with permission.[ 76 ] Copyright 2020, WILEY‐VCH. d) I–V characteristics of Ag/BA2CsAgBiBr7/Pt. e,f) I–V characteristics of the device operated at 85 °C and for 22 days. Reprinted with permission.[ 77 ] Copyright 2021, The Royal Society of Chemistry.
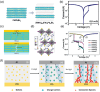
a) Schematic of FAPbBr3 and (RNH3)2(FA)1PbBr7 molecular structures. b) I−V curve of quasi‐2D (RNH3)2(FA)1Pb2Br7 with ligand concentrations of 0.8 vol %. c) Illustration of the formation of VBr filaments. Reprinted with permission.[ 86 ] Copyright 2021, American Chemical Society. d) Crystal structure of MA2PbI2(SCN)2 and orientation. e) Typical I–V curve of MA2PbI2(SCN)2‐based memory devices. f) Schematic diagram of MA2PbI2(SCN)2‐based memory mechanism. Reprinted with permission.[ 87 ] Copyright 2018, Wiley‐VCH.
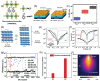
a) Schematic illustration of (3AMP)PbI4. b) Schematic demonstration of the grain size of (3AMP)PbI4 films under different volume ratios. c) Statistical distribution of ON/OFF ratio under different V DMF: V DMSO. Reprinted with permission.[ 88 ] Copyright 2022, American Chemical Society. d) Schematic illustration of 2D RP perovskite formation process. e,f) I−V curves of devices fabricated by e) MAPbI3−xClx and f) 2D/3D heterostructure structure. Reprinted with permission.[ 89 ] Copyright 2020, American Chemical Society. g) Endurance behavior measured with the DC pulses for 2D and 2D/3D RS devices. h) Thermal conductivity of 2D and 3D perovskite films. i) Graph of the COMSOL simulation of the 2D/3D structure. Reprinted with permission.[ 64 ] Copyright 2020, American Chemical Society.
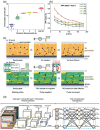
a) EPSC of MHPs artificial synapses with different dimensionality. b) PPF index (A2/A1) with time interval from 20 to 180 ms. c) Schematic mechanism of ion migration in artificial synapses. Top:3D, Bottom:quasi‐2D. Reprinted with permission.[ 41 ] Copyright 2019, Wiley‐VCH. d) Memristor device consist of CIFAR‐10 dataset and a convolutional neural network. Reprinted with permission.[ 78 ] Copyright 2022, Wiley‐VCH.
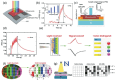
a) Schematic of the device structure based on (PEA)2SnI4. b) ΔEPSC under 10 light pulses. The inset shows the frequency‐dependent EPSC gain (A10/A1). Reprinted with permission.[ 79 ] Copyright 2018, The Royal Society of Chemistry. c) Schematic illustration of the (PEA)2SnI4 artificial synaptic devices. The insets show the input light spikes and the output PSC. d) The ΔPSC triggered by a train of 10 light spikes (20 ms, 20 Hz). Reprinted with permission.[ 96 ] Copyright 2019, Wiley‐VCH. e) Simulation process of the synaptic transistor's recognition. f) 12 × 5 transistor arrays of LED light. Input images in the letter “G,” “o,” “o,” and “d” consisting of four 3 × 5 pixels encoded by red, green, blue, and NIR light, respectively. Reprinted with permission.[ 97 ] Copyright 2021, Wiley‐VCH. g) Current response before stimulus, at the last stimulus, and 300 s after last stimulus by inputting letter of “N”. Reprinted with permission.[ 98 ] Copyright 2021, The Royal Society of Chemistry.
Similar articles
-
Halide Perovskites for Memristive Data Storage and Artificial Synapses.
Kwak KJ, Lee DE, Kim SJ, Jang HW. Kwak KJ, et al. J Phys Chem Lett. 2021 Sep 23;12(37):8999-9010. doi: 10.1021/acs.jpclett.1c02332. Epub 2021 Sep 13. J Phys Chem Lett. 2021. PMID: 34515487
-
Ganaie MM, Bravetti G, Sahu S, Kumar M, Milić JV. Ganaie MM, et al. Mater Adv. 2024 Jan 3;5(5):1880-1886. doi: 10.1039/d3ma00618b. eCollection 2024 Mar 4. Mater Adv. 2024. PMID: 38444935 Free PMC article.
-
Halide Perovskites: A New Era of Solution-Processed Electronics.
Younis A, Lin CH, Guan X, Shahrokhi S, Huang CY, Wang Y, He T, Singh S, Hu L, Retamal JRD, He JH, Wu T. Younis A, et al. Adv Mater. 2021 Jun;33(23):e2005000. doi: 10.1002/adma.202005000. Epub 2021 May 3. Adv Mater. 2021. PMID: 33938612 Review.
-
Loizos M, Rogdakis K, Luo W, Zimmermann P, Hinderhofer A, Lukić J, Tountas M, Schreiber F, Milić JV, Kymakis E. Loizos M, et al. Nanoscale Horiz. 2024 Jun 24;9(7):1146-1154. doi: 10.1039/d4nh00104d. Nanoscale Horiz. 2024. PMID: 38767026 Free PMC article.
-
Two-Dimensional Materials for Halide Perovskite-Based Optoelectronic Devices.
Chen S, Shi G. Chen S, et al. Adv Mater. 2017 Jun;29(24). doi: 10.1002/adma.201605448. Epub 2017 Mar 3. Adv Mater. 2017. PMID: 28256781 Review.
Cited by
-
Optical Bio-Inspired Synaptic Devices.
Li P, Wang K, Jiang S, He G, Zhang H, Cheng S, Li Q, Zhu Y, Fu C, Wei H, He B, Li Y. Li P, et al. Nanomaterials (Basel). 2024 Sep 29;14(19):1573. doi: 10.3390/nano14191573. Nanomaterials (Basel). 2024. PMID: 39404300 Free PMC article. Review.
-
Kim SY, Zhang H, Rubio-Magnieto J. Kim SY, et al. J Phys Chem Lett. 2024 Oct 10;15(40):10087-10103. doi: 10.1021/acs.jpclett.4c02170. Epub 2024 Sep 27. J Phys Chem Lett. 2024. PMID: 39331473 Free PMC article. Review.
References
-
- Ma L. P., Liu J., Yang Y., Appl. Phys. Lett. 2002, 80, 2997.
-
- Waser R., Dittmann R., Staikov G., Szot K., Adv. Mater. 2009, 21, 2632. - PubMed
-
- Gao S., Yi X., Shang J., Liu G., Li R. W., Chem. Soc. Rev. 2018, 48, 1531. - PubMed
-
- Wang Z., Wu H., Burr G. W., Hwang C. S., Wang K. L., Xia Q., Yang J. J., Nat. Rev. Mater. 2020, 5, 173.
-
- Betal A., Bera J., Sahu S., J. Mater. Chem. C 2023, 11, 4674.
Publication types
Grants and funding
- 2021M690127/China Postdoctoral Science Foundation
- 23A0636/Scientific Research Fund of Hunan Provincial Education Department
- 2021JJ40141/Natural Science Foundation of Hunan Province
- kq2107023/Training Project of Changsha City for Distinguished Young Scholars
- 2023-JC-QN-0680/Shaanxi Natural Science Foundation of China
LinkOut - more resources
Full Text Sources