Nanoengineered Silica-Based Biomaterials for Regenerative Medicine - PubMed
- ️Mon Jan 01 2024
Review
Nanoengineered Silica-Based Biomaterials for Regenerative Medicine
Mohamed A A Abdelhamid et al. Int J Mol Sci. 2024.
Abstract
The paradigm of regenerative medicine is undergoing a transformative shift with the emergence of nanoengineered silica-based biomaterials. Their unique confluence of biocompatibility, precisely tunable porosity, and the ability to modulate cellular behavior at the molecular level makes them highly desirable for diverse tissue repair and regeneration applications. Advancements in nanoengineered silica synthesis and functionalization techniques have yielded a new generation of versatile biomaterials with tailored functionalities for targeted drug delivery, biomimetic scaffolds, and integration with stem cell therapy. These functionalities hold the potential to optimize therapeutic efficacy, promote enhanced regeneration, and modulate stem cell behavior for improved regenerative outcomes. Furthermore, the unique properties of silica facilitate non-invasive diagnostics and treatment monitoring through advanced biomedical imaging techniques, enabling a more holistic approach to regenerative medicine. This review comprehensively examines the utilization of nanoengineered silica biomaterials for diverse applications in regenerative medicine. By critically appraising the fabrication and design strategies that govern engineered silica biomaterials, this review underscores their groundbreaking potential to bridge the gap between the vision of regenerative medicine and clinical reality.
Keywords: biocompatibility; biomedical imaging; biomimetic scaffolds; nanoengineered silica; regenerative medicine; stem cell therapy; targeted drug delivery; tissue engineering.
Conflict of interest statement
The authors declare no conflicts of interest.
Figures
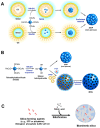
(A) Microemulsion, (B) Stöber, and (C) biomimetic approaches for silica nanoparticle synthesis. (F127: Pluronic F127 block copolymer surfactant; CTAB: cetyltrimethylammonium bromide). (A,B): Reproduced with permission from [33], copyright 2020 Frontiers (CC BY 4.0 DEED).
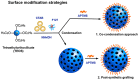
Surface modification strategies for silica nanoparticles. (F127: Pluronic F127 block copolymer surfactant; CTAB: cetyltrimethylammonium bromide; APTMS: [3-aminopropyl]trimethoxysilane). Reproduced with permission from [33], copyright 2020 Frontiers (CC BY 4.0 DEED).
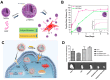
Mesoporous silica nanoparticles for targeted drug delivery. (A) Fabrication and in vitro cellular interactions of peptide-loaded MSNs (p-MSNs). (B) BFP release kinetics. (Inset: 10 h magnification). (C) Schematic depicting targeted delivery, penetration, and triggered release of PEOz-iRGD-modified MSNs in tumor cells. (D) Evaluation of tumor growth inhibition post-intravenous injection. (A,B): Reproduced with permission from [98], copyright 2015 Elsevier B.V. (C,D): Reproduced with permission from [99], copyright 2024 Elsevier B.V (CC BY-NC-ND 4.0 DEED).
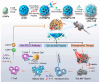
Targeted drug delivery for spinal tumor therapy. Schematic of a nanocarrier delivering a combo therapy (photosensitizer, peptide vaccine, PD-L1 inhibitor) for spinal tumors via PDT and immune stimulation. (UCMS: Upconverting nanoparticle core encapsulated in mesoporous silica shell). Reproduced with permission from [100], copyright 2021 Springer Nature (CC BY 4.0 DEED).
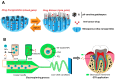
Biopolymer-enhanced drug delivery with silica nanoparticles. (A) pH-triggered drug release: a gatekeeper system controls drug release from silica based in acidic environments. (B) Dual drug delivery for bone regeneration: core–shell nanofibers deliver growth factors and antibiotics for improved bone healing. (A): Reproduced with permission from [102], copyright 2016 Elsevier B.V. (B): Reproduced with permission from [103], copyright 2020 American Chemical Society.
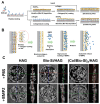
Biomimetic scaffolds for enhanced bone regeneration. (A) Schematic of HA core scaffold preparation with collagen layering and Ectp1-mediated silica deposition for various configurations. (B) Schematic depicting growth factor (e.g., BMP2) adsorption and release on (Col/Bio-Si)2/HA scaffold surface. (C) µCT images (transverse and coronal) of rat calvarial bone regeneration after implantation with scaffolds (without or with BMP2). (A–C): Reproduced with permission from [15], copyright 2023 Elsevier B.V.
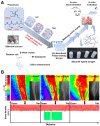
Fabrication and bone formation evaluation of silica–silk fibroin aerogel scaffolds. (A) Schematic of silica–silk fibroin (SF) aerogel scaffold synthesis using sol–gel reaction, unidirectional freeze-casting, and supercritical drying. (B) Radiographic analysis with color-coded bone density based on radiographs (lower right). Red indicates high bone density, green represents minimal bone formation. Black line marks quantified bone area. (A,B): Reproduced with permission from [128], copyright 2019 American Chemical Society.
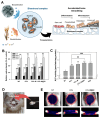
Biomineral complex for bone regeneration. (A) Schematic of the complex promoting surface bone formation. Evaluation of mesenchymal stem cell (MSC) behavior. (B) Cell proliferation at 72 h. (C) ALP activity after 5 days. Significant differences were observed between groups (## p < 0.01; ***, ###p < 0.005). (D) Implantation of coccolith-based bone substitute. (E) Micro-CT images show bone regeneration over 6 weeks. Newly formed bone in red, defect areas in blue (cross-sectional and sagittal views). (A–E): Reproduced with permission from [108], copyright 2021 American Chemical Society.
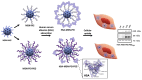
Schematic illustration of the process of human serum albumin (HSA) adsorption and cellular uptake of MSN-PEI nanocomposites by stem cells. Reproduced with permission from [16], copyright 2020 Elsevier B.V.
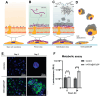
Bioinspired silicification approach for engineering hASC surface. (A) Cell membrane bare surface, negatively charged. (B) Primer step. Chitosan–carnitine (CHT-CAR) interacts with cell membrane, forming a positively charged layer. (C) Biosilicification step and (D) detached cells with partial coating, forming hASCs@SiO2BP. (E) Cell viability assay at days 1 and 7. Live cells (blue), dead cells (red), silica (green). (F) Metabolic activity at 0 and 24 h. **** p < 0.0001, n.s, not significant. (A–F): Reproduced with permission from [110], copyright 2021 Wiley-VCH GmbH.
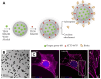
(A) Schematic of the two-cycle Stöber method for synthesizing dual-color silica nanoparticles. (B) TEM image of silica NPs (49 ± 3 nm). (C) Comparison of wide-field and Lattice SIM2 imaging of MCF7 cells after incubation with functionalized silica NPs. The inset provides a close-up view of the region marked by the dotted white squares. White arrows highlight features better resolved with Lattice SIM2 compared to wide-field imaging. (A–C): Reproduced with permission from [112], copyright 2023 Royal Society of Chemistry (CC BY 3.0 DEED).
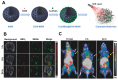
(A) Schematic of MDNs formation. In vivo visualization of MDNs post intravenous administration. (B) Photoacoustic imaging at different time points, demonstrating selective tumor enhancement (yellow dashed circle). (C) PET imaging shows continuous tumor uptake enhancement (yellow arrow), with the bladder area marked (white dashed circle). (A–C): Reproduced with permission from [117], copyright 2017 WILEY-VCH Verlag GmbH & Co. KGaA, Weinheim.
Similar articles
-
Smart Porous Multi-Stimulus Polysaccharide-Based Biomaterials for Tissue Engineering.
Alvarado-Hidalgo F, Ramírez-Sánchez K, Starbird-Perez R. Alvarado-Hidalgo F, et al. Molecules. 2020 Nov 13;25(22):5286. doi: 10.3390/molecules25225286. Molecules. 2020. PMID: 33202707 Free PMC article. Review.
-
Biomolecule delivery to engineer the cellular microenvironment for regenerative medicine.
Bishop CJ, Kim J, Green JJ. Bishop CJ, et al. Ann Biomed Eng. 2014 Jul;42(7):1557-72. doi: 10.1007/s10439-013-0932-1. Epub 2013 Oct 30. Ann Biomed Eng. 2014. PMID: 24170072 Free PMC article. Review.
-
Bello AB, Kim D, Kim D, Park H, Lee SH. Bello AB, et al. Tissue Eng Part B Rev. 2020 Apr;26(2):164-180. doi: 10.1089/ten.TEB.2019.0256. Epub 2020 Feb 4. Tissue Eng Part B Rev. 2020. PMID: 31910095 Review.
-
The Potential Application of Biomaterials in Cardiac Stem Cell Therapy.
Sahito RG, Sureshkumar P, Sotiriadou I, Srinivasan SP, Sabour D, Hescheler J, Pfannkuche K, Sachinidis A. Sahito RG, et al. Curr Med Chem. 2016;23(6):589-602. doi: 10.2174/092986732306160303151041. Curr Med Chem. 2016. PMID: 26951086 Review.
-
Oligoaniline-based conductive biomaterials for tissue engineering.
Zarrintaj P, Bakhshandeh B, Saeb MR, Sefat F, Rezaeian I, Ganjali MR, Ramakrishna S, Mozafari M. Zarrintaj P, et al. Acta Biomater. 2018 May;72:16-34. doi: 10.1016/j.actbio.2018.03.042. Epub 2018 Apr 4. Acta Biomater. 2018. PMID: 29625254 Review.
Cited by
-
Aaddouz M, El Yousfi R, Sabbahi R, Azzaoui K, Yahyaoui MI, Asehraou A, Hammouti B, Laoutid F, Alanazi MM, Mejdoubi E. Aaddouz M, et al. Molecules. 2024 Jul 25;29(15):3483. doi: 10.3390/molecules29153483. Molecules. 2024. PMID: 39124887 Free PMC article.
References
-
- Bayon Y., Vertès A.A., Ronfard V., Egloff M., Snykers S., Salinas G.F., Thomas R., Girling A., Lilford R., Clermont G. Translating cell-based regenerative medicines from research to successful products: Challenges and solutions. Tissue Eng. Part B Rev. 2014;20:246–256. doi: 10.1089/ten.teb.2013.0727. - DOI - PubMed
Publication types
MeSH terms
Substances
Grants and funding
LinkOut - more resources
Full Text Sources
Miscellaneous