Unravelling long-term impact of water abstraction and climate change on endorheic lakes: A case study of Shortandy Lake in Central Asia - PubMed
- ️Mon Jan 01 2024
Unravelling long-term impact of water abstraction and climate change on endorheic lakes: A case study of Shortandy Lake in Central Asia
Marzhan Baigaliyeva et al. PLoS One. 2024.
Abstract
Endorheic lakes, lacking river outflows, are highly sensitive to environmental changes and human interventions. Central Asia (CA) has over 6000 lakes that have experienced substantial water level variability in the past century, yet causes of recent changes in many lakes remain unexplored. Modelling hydrological processes for CA lakes poses challenges in separating climatic change impacts from human management impacts due to limited data and long-term variability in hydrological regimes. This study developed a spatially lumped empirical model to investigate the effects of climate change and human water abstraction, using Shortandy Lake in Burabay National Nature Park (BNNP) as a case study. Modelling results show a significant water volume decline from 231.7x106m3 in 1986 to 172.5x106m3 in 2016, primarily driven by anthropogenic water abstraction, accounting for 92% of the total volume deficit. The highest rates of water abstraction (greater than 25% of annual outflow) occurred from 1989 to 1993, coinciding with the driest period. Since 2013, the water volume has increased due to increased precipitation and, more importantly, reduced water abstraction. Despite limited observational data with which to calibrate the model, it performs well. Our analysis underscores the challenges in modelling lakes in data-sparse regions such as CA, and highlights the importance and benefits of developing lake water balance models for the region.
Copyright: © 2024 Baigaliyeva et al. This is an open access article distributed under the terms of the Creative Commons Attribution License, which permits unrestricted use, distribution, and reproduction in any medium, provided the original author and source are credited.
Conflict of interest statement
The authors have declared that no competing interests exist
Figures
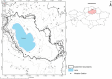
The administrative boundary shapefile of Kazakhstan and its districts is obtained from an open license source known as the geoBoundaries Global Administrative Database [37].
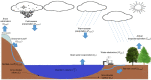
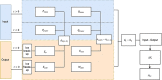
Prain is rainfall, Psnow is snow, Qrain is rainfall-generated runoff, Qsnow is snowmelt runoff and Gi−Go is a groundwater flux, Acatch is Shortandy Lake catchment area, AL(i-1) is the lake area of the previous month, Eo is lake evaporation, Wabs is total water abstraction from surface and groundwater combined, Eact is evapotranspiration from the catchment excluding Eo, Esub is sublimation, ΔVi is water volume change, ALi is lake area corresponding to ΔVi, and t is mean air temperature.
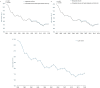
(a) volume simulated by the regional groundwater model, (b) volume simulated by groundwater flux estimated by water balance approach, and (c) water volume dynamics estimated by Eq 1, where errors bars show standard deviation of the lake volume obtained from a one-by-one sensitivity analysis with four selected model parameters.
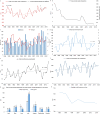
(a) Total annual open water evaporation estimated by the simplified Penman equation and actual evapotranspiration by SSEBop is the actual evapotranspiration for the lake by Operational Simplified Surface Energy Balance model, (b) Total annual surface and groundwater abstraction, (c) Total annual snow (Psnow) and mean temperature during snowmelt, (d) Total seasonal snowmelt runoff, (e) Total annual snow sublimation, (f) Total annual rainfall in the catchment, (g) Total monthly rainfall distribution and total monthly rainfall distribution in wet years (excessive rainfall events), and error bars show a standard deviation, (h) Total monthly rainfall-runoff produced in the catchment.
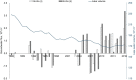
(ii) is estimated using the Shortandy water balance model in Eq 1.
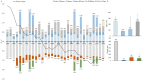
(a) Total annual input and output variables (mm) (b) Relative contribution and mean values of input and output variables from 1986 to 2016, where error bars show standard deviation.
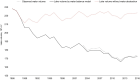
The grey line shows water volume estimated by measured lake levels, the black line is the lake volume simulated by the water balance model, and the red line shows the water volume changes without water abstraction.
Similar articles
-
Wang J, Liu D, Tian S, Ma J, Wang L. Wang J, et al. Sci Rep. 2022 Apr 20;12(1):6535. doi: 10.1038/s41598-022-10284-y. Sci Rep. 2022. PMID: 35443769 Free PMC article.
-
Divergent change patterns observed in hydrological fluxes entering China's two largest lakes.
Li T, Liao Q, Wang S, Fu B. Li T, et al. Sci Total Environ. 2022 Apr 15;817:152969. doi: 10.1016/j.scitotenv.2022.152969. Epub 2022 Jan 10. Sci Total Environ. 2022. PMID: 35026261
-
Changes in the area of inland lakes in arid regions of central Asia during the past 30 years.
Bai J, Chen X, Li J, Yang L, Fang H. Bai J, et al. Environ Monit Assess. 2011 Jul;178(1-4):247-56. doi: 10.1007/s10661-010-1686-y. Epub 2010 Sep 10. Environ Monit Assess. 2011. PMID: 20830516
-
Porcal P, Koprivnjak JF, Molot LA, Dillon PJ. Porcal P, et al. Environ Sci Pollut Res Int. 2009 Sep;16(6):714-26. doi: 10.1007/s11356-009-0176-7. Epub 2009 May 22. Environ Sci Pollut Res Int. 2009. PMID: 19462191 Review.
-
A review on the research progress of lake water volume estimation methods.
An C, Zhang F, Chan NW, Johnson VC, Shi J. An C, et al. J Environ Manage. 2022 Jul 15;314:115057. doi: 10.1016/j.jenvman.2022.115057. Epub 2022 Apr 19. J Environ Manage. 2022. PMID: 35452887 Review.
References
-
- Chen F. Holocene moisture evolution in arid central Asia and its out-of-phase relationship with Asian monsoon history. Quaternary Science Reviews. 2008;27:351–64.
-
- Salnikov V, Turulina G, Polyakova S, Petrova Y, Skakova A. Climate change in Kazakhstan during the past 70 years. Quaternary International. 2015;358:77–82.
-
- Petr T. Lake Balkhash, Kazakhstan. International Journal of Salt Lake Research. 1992;1(1):21–46.
-
- Micklin P. The Aral Sea Disaster. The annual Review of Earth and Planetary Sciences. 2007;35:47–72.
-
- Chiba T, Endo K, Sugai T, Haraguchi T, Kondo R, Kubota J. Reconstruction of Lake Balkhash levels and precipitation/evaporation changes during the last 2000 years from fossil diatom assemblages. Quaternary International. 2016;397:330–41.
MeSH terms
Substances
Grants and funding
The authors received no specific funding for this work.
LinkOut - more resources
Full Text Sources
Medical
Miscellaneous