Targeting Asparagine Metabolism in Well-Differentiated/Dedifferentiated Liposarcoma - PubMed
- ️Mon Jan 01 2024
. 2024 Aug 30;16(17):3031.
doi: 10.3390/cancers16173031.
Blake R Wilde 2 3 4 , Danielle S Graham 1 2 , Serena Lofftus 1 2 , Tyler McCaw 1 2 , Nedas Matulionis 3 4 , Sarah M Dry 2 5 , Joseph G Crompton 1 2 , Fritz C Eilber 1 2 , Thomas G Graeber 2 6 , David B Shackelford 2 7 , Heather R Christofk 2 3 4 , Brian E Kadera 1 2
Affiliations
- PMID: 39272889
- PMCID: PMC11394161
- DOI: 10.3390/cancers16173031
Targeting Asparagine Metabolism in Well-Differentiated/Dedifferentiated Liposarcoma
Kyle D Klingbeil et al. Cancers (Basel). 2024.
Abstract
Background: mTORC1 activity is dependent on the presence of micronutrients, including Asparagine (Asn), to promote anabolic cell signaling in many cancers. We hypothesized that targeting Asn metabolism would inhibit tumor growth by reducing mTORC1 activity in well-differentiated (WD)/dedifferentiated (DD) liposarcoma (LPS).
Methods: Human tumor metabolomic analysis was utilized to compare abundance of Asn in WD vs. DD LPS. Gene set enrichment analysis (GSEA) compared relative expression among metabolic pathways upregulated in DD vs. WD LPS. Proliferation assays were performed for LPS cell lines and organoid models by using the combination treatment of electron transport chain (ETC) inhibitors with Asn-free media. 13C-Glucose-labeling metabolomics evaluated the effects of combination treatment on nucleotide synthesis. Murine xenograft models were used to assess the effects of ETC inhibition combined with PEGylated L-Asparaginase (PEG-Asnase) on tumor growth and mTORC1 signaling.
Results: Asn was enriched in DD LPS compared to WD LPS. GSEA indicated that mTORC1 signaling was upregulated in DD LPS. Within available LPS cell lines and organoid models, the combination of ETC inhibition with Asn-free media resulted in reduced cell proliferation. Combination treatment inhibited nucleotide synthesis and promoted cell cycle arrest. In vivo, the combination of ETC inhibition with PEG-Asnase restricted tumor growth.
Conclusions: Asn enrichment and mTORC1 upregulation are important factors contributing to WD/DD LPS tumor progression. Effective targeting strategies require limiting access to extracellular Asn and inhibition of de novo synthesis mechanisms. The combination of PEG-Asnase with ETC inhibition is an effective therapy to restrict tumor growth in WD/DD LPS.
Keywords: ATF4; asparaginase; asparagine metabolism; complex I inhibitor; electron transport chain; mTORC1 signaling; patient-derived organoids; patient-derived xenograft; well-differentiated/dedifferentiated liposarcoma.
Conflict of interest statement
The authors declare no conflicts of interest.
Figures
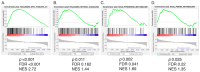
mTORC1 Signaling Among Top Gene Sets Enriched in DD LPS. Of the 288 metabolic pathway-related gene sets analyzed by GSEA, the top four ranked gene sets by NES are displayed. Gene sets were analyzed based on enrichment of DD LPS (n = 18) compared to WD LPS (n = 29). p < 0.05 and FDR (q) < 0.25 were used as cutoffs for significance. (A) Hallmark Myc Targets: NES 2.72, FDR < 0.001, p < 0.001, (B) Hallmark mTORC1 Signaling: NES 1.44, FDR 0.162, p = 0.011, (C) KEGG pyrimidine metabolism: NES 1.69, FDR 0.041, p = 0.002, and (D) KEGG purine metabolism: NES 1.35, FDR 0.22, p = 0.025.
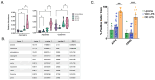
Increased Asparagine Abundance and Upregulated de novo Synthesis in DD LPS. (A) Human LPS samples freshly harvested from surgical resection and stored in liquid nitrogen underwent metabolomic analysis to compare relative abundance of key metabolites. Asn was found to be significantly more abundant among both WD LPS (p = 0.040) and DD LPS (p = 0.019) compared to adjacent normal adipose tissue. Additionally, Asn was significantly more abundant among DD LPS samples compared to WD LPS (p = 0.035). Each dot represents a unique patient sample. (B) Tabular format of metabolite comparisons between WD and DD LPS, ordered by p-value. Listed are the top 10 metabolites that significantly differed between WD and DD LPS. Notably, asparagine was ranked within the top four metabolites. (C) A tissue microarray was used to compare expression of de novo synthesis enzymes, ASNS and ATF4, among lipomatous tumors. IHC staining was quantified by cell positivity per tissue core. DD LPS was found to have significantly higher ATF4 cell positivity compared to WD LPS (p < 0.001) and ASNS cell positivity compared to WD LPS (p < 0.0001). Lipoma is a benign adipose tumor used as a control comparator. Each dot represents a unique patient sample. * p < 0.05, *** p < 0.001, **** p < 0.0001. ns = not significant.
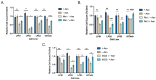
WD/DD LPS Display Sensitivity to Asparagine Depletion in vitro. Cell lines included DD LPS (LPS1, LPS2 and LPS3) and WD LPS (93T449). Cells were treated with media containing dialyzed FBS with supplemented 0.1 mM Asn (+Asn) or DMSO (−Asn). Cells were then treated with specified drug treatments for 48 h followed by quantification. (A) Metformin (2.5 mM) treatment combined with −Asn led to the greatest reduction in cell count compared to control. (B) Rotenone (25 nM), combined with −Asn treatment similarly resulted in the greatest reduction in cell count compared to control. (C) IACS (25 nM) demonstrated similar results. All +Asn vs −Asn/ETCi comparisons were significant with p < 0.001. * p < 0.05, ** p < 0.01, *** p < 0.001, **** p < 0.0001. ns = not significant.
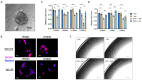
WD/DD LPS PDTOs Display Similar Sensitivity to Asparagine Depletion. PDTOs were generated following patient tumor harvest and grown in pyruvate-free DMEM with 10% v/v dialyzed FBS supplemented with 0.1 mM of Asn (+Asn) or DMSO (−Asn). Organoids were allowed to develop over 3–5 days prior to 72 h treatment with 50 nM IACS. (A) Representative PDTO viewed under light microscopy, scale bar 50 μm. (B) FISH analysis of PDTO’s using MDM2 amplification as a marker of LPS cell validation. Magenta color denotes MDM2 gene. Blue color denotes nuclear staining. Both WD and DD LPS PDTO samples demonstrate high amplification of MDM2, scale bar 20 μm. (C) WD LPS PDTOs, demonstrating combined 50 nM IACS/−Asn therapy, promotes the greatest reduction in organoid growth. LPS053 in particular was found to have a profound sensitivity to combined therapy. Each dot represents a technical replicate. (D) DD LPS PDTOs, demonstrating similar effects across all samples, in which combined IACS/−Asn therapy promotes the greatest reduction in organoid growth. Each dot represents a technical replicate. (E) LPS2 maxi-ring organoids treated with 72 h of 50 nM of IACS or DMSO (+/−Asn) were imaged under brightfield microscopy. Compared to +Asn/DMSO control, -Asn/IACS conditions demonstrated a lower total cell count and attenuated formation of organoid clusters, scale bar 251 μm. * p < 0.05, ** p < 0.01, *** p < 0.001, **** p < 0.0001. ns = not significant.
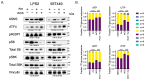
Asparagine Depletion Impairs mTORC1 Activity and Nucleotide Biosynthesis. (A) Western blots of LPS2 and 93T449 lysates 24 h post-treatment of 25 nM of IACS or DMSO in the absence or presence of 0.1 mM of Asn. Lysates were immunoblotted for ASNS, ATF4 and mTORC1 activation markers (pS6K, total S6K, pS6, total S6, p4EBP1). Vinculin was used as a loading control. (B) Fractional contributions of the various U-13C-glucose nucleotide isotopomers following 24 h treatment of 25 nM of IACS or DMSO in the absence or presence of 0.1 mM of Asn. The m + 0 isotopomer denotes lack of incorporation of the U-13C-glucose carbons into the specified nucleotide. The fraction of m+ > 0 denotes the rate of nucleotide biosynthesis. The combination of IACS and −Asn reduced the biosynthesis of all four nucleotides. **** p < 0.0001.
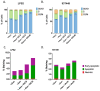
Asparagine Depletion Induces Cell Cycle Arrest and Apoptosis. (A) LPS2 and (B) 93T449 cells were treated with 25 nM of IACS or DMSO in the presence or absence of 0.1 mM of Asn for 24 h, followed by processing for FACS analysis to assess whether combination treatments inhibit cell growth by inducing cell cycle arrest (increasing fraction of cells in G0/G1 phase). A three-hour treatment with Staurosporine 100 nM was used as a positive control for cell cycle arrest. For both LPS2 and 93T449, combination treatment resulted in the highest fraction of cells in G0/G1 phase, indicating that cell cycle arrest does contribute to growth inhibition. This effect is partially reversed in the presence of Asn for both cell lines. (C) LPS2 and (D) 93T449 cells were similarly treated with 25 nM of IACS or DMSO in the presence or absence of 0.1 mM of Asn for 24 h, followed by processing for FACS analysis to assess whether combination treatments inhibit cell growth by inducing apoptosis. For both LPS2 and 93T449, combination treatment resulted in the highest rates of apoptosis, which was partially reversed in the presence of Asn.
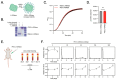
Synthesis and Validation of PEGylated Asparaginase for in vivo Drug Trials. (A) Schematic of the PEGylation design to improve stability in serum. (B) SDS-PAGE gel identifying the fractions of drug that contain PEG-ASNase following PEGylation. (C) Catalytic activity of PEG-ASNase was compared to the unmodified ASNase using a colorimetric Asparaginase assay and displayed similar activity rates. (D) Total amount of Asn consumed at the endpoint of the Asparaginase assay demonstrating no difference in activity after PEGylation. (E) Schematic of the experiment design, wherein PEG-ASNase was delivered to mice via tail vein injection at 0, 20, 50, 150 and 300 units/kg. Blood was collected by retroorbital bleeding prior to injection and 1, 7 and 10 days following injection. Plasma was isolated from the blood and analyzed for asparagine and glutamine using LC-MS. (F) Relative Asn levels from mice serum at the specified treatment dosing (mean ± SD). The lowest effective dose was 20 units/kg, which depleted Asn levels to at least 10 days. Treatment did not affect Gln levels, which is a known off-target effect of ASNase.
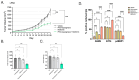
Combination of Metformin and PEG-ASNase Impairs Tumor Growth. (A) LPS2 tumor xenograft growth curves from treatment start date through endpoint. The combination of metformin with PEG-ASNase resulted in significant impairment of tumor growth compared to control (p < 0.0001). (B) Tumor volume at the study endpoint. Each dot represents an individual tumor xenograft. (C) Tumor mass at the study endpoint. Each dot represents an individual tumor xenograft. (D) IHC was performed on formalin-fixed tumor tissue and quantified by cell positivity/sample. Samples were probed for ASNS, ATF4 and the marker of mTORC1 activation (p4EBP1). ASNS and ATF4 positivity was significantly higher in the Met/PEG-ASNase group compared to controls. mTORC1 activity was also attenuated as shown by loss of p4EBP1 staining in the Met/PEG-ASNase group compared to controls. * p < 0.05, *** p < 0.001, **** p < 0.0001. ns = not significant.
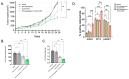
Combination of IACS and PEG-ASNase Impairs Tumor Growth. (A) LPS2 tumor xenograft growth curves from treatment start date through endpoint. The combination of IACS with PEG-ASNase resulted in significant impairment of tumor growth compared to control (p < 0.0001). IACS as monotherapy also resulted in a significant impairment of tumor growth (p < 0.01). (B) Tumor volume at the study endpoint. Each dot represents an individual tumor xenograft. (C) Tumor mass at the study endpoint. Each dot represents an individual tumor xenograft. (D) Quantified IHC of formalin-fixed tumor tissue probed for ASNS, ATF4 and a marker of mTORC1 activation (p4EBP1). ASNS and ATF4 expression was significantly higher in the IACS/PEG-ASNase group compared to controls. p4EBP1 expression was also attenuated in the Met/PEG-ASNase group compared to controls. IACS monotherapy resulted in a loss of p4EBP1, although did not affect ATF4 o ASNS expression. ** p < 0.01, *** p < 0.001, **** p < 0.0001. ns = not significant.
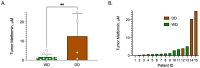
Metformin Concentration in Tumor Samples of Patients taking Metformin. Patients who were taking metformin at the time of surgical resection were included. Metformin concentrations were measured from cryobanked WD/DD LPS tissue samples (n = 15) using MS/LC. (A) Bar graph comparing mean ± SD metformin concentration by histologic group, WD vs. DD, noting that DD displayed significantly higher metformin compared to WD, p = 0.0074. Each dot represents a unique patient tumor sample. (B) Bar graph of tumor metformin concentration from each patient sample, noting high inter-subject variability. ** p < 0.01.
Similar articles
-
Elwenspoek MM, Thom H, Sheppard AL, Keeney E, O'Donnell R, Jackson J, Roadevin C, Dawson S, Lane D, Stubbs J, Everitt H, Watson JC, Hay AD, Gillett P, Robins G, Jones HE, Mallett S, Whiting PF. Elwenspoek MM, et al. Health Technol Assess. 2022 Oct;26(44):1-310. doi: 10.3310/ZUCE8371. Health Technol Assess. 2022. PMID: 36321689 Free PMC article.
-
Harnan S, Kearns B, Scope A, Schmitt L, Jankovic D, Hamilton J, Srivastava T, Hill H, Ku CC, Ren S, Rothery C, Bojke L, Sculpher M, Woods B. Harnan S, et al. Health Technol Assess. 2024 Oct;28(73):1-230. doi: 10.3310/YAPL9347. Health Technol Assess. 2024. PMID: 39487661 Free PMC article.
-
Antioxidants for female subfertility.
Showell MG, Mackenzie-Proctor R, Jordan V, Hart RJ. Showell MG, et al. Cochrane Database Syst Rev. 2020 Aug 27;8(8):CD007807. doi: 10.1002/14651858.CD007807.pub4. Cochrane Database Syst Rev. 2020. PMID: 32851663 Free PMC article.
-
Antioxidants for female subfertility.
Showell MG, Mackenzie-Proctor R, Jordan V, Hart RJ. Showell MG, et al. Cochrane Database Syst Rev. 2017 Jul 28;7(7):CD007807. doi: 10.1002/14651858.CD007807.pub3. Cochrane Database Syst Rev. 2017. PMID: 28752910 Free PMC article. Updated. Review.
-
Antibody tests for identification of current and past infection with SARS-CoV-2.
Fox T, Geppert J, Dinnes J, Scandrett K, Bigio J, Sulis G, Hettiarachchi D, Mathangasinghe Y, Weeratunga P, Wickramasinghe D, Bergman H, Buckley BS, Probyn K, Sguassero Y, Davenport C, Cunningham J, Dittrich S, Emperador D, Hooft L, Leeflang MM, McInnes MD, Spijker R, Struyf T, Van den Bruel A, Verbakel JY, Takwoingi Y, Taylor-Phillips S, Deeks JJ; Cochrane COVID-19 Diagnostic Test Accuracy Group. Fox T, et al. Cochrane Database Syst Rev. 2022 Nov 17;11(11):CD013652. doi: 10.1002/14651858.CD013652.pub2. Cochrane Database Syst Rev. 2022. PMID: 36394900 Free PMC article. Review.