Hyperexpansion of genetic diversity and metabolic capacity of extremophilic bacteria and archaea in ancient Andean lake sediments - PubMed
- ️Mon Jan 01 2024
Hyperexpansion of genetic diversity and metabolic capacity of extremophilic bacteria and archaea in ancient Andean lake sediments
María Ángeles Lezcano et al. Microbiome. 2024.
Abstract
Background: The Andean Altiplano hosts a repertoire of high-altitude lakes with harsh conditions for life. These lakes are undergoing a process of desiccation caused by the current climate, leaving terraces exposed to extreme atmospheric conditions and serving as analogs to Martian paleolake basins. Microbiomes in Altiplano lake terraces have been poorly studied, enclosing uncultured lineages and a great opportunity to understand environmental adaptation and the limits of life on Earth. Here we examine the microbial diversity and function in ancient sediments (10.3-11 kyr BP (before present)) from a terrace profile of Laguna Lejía, a sulfur- and metal/metalloid-rich saline lake in the Chilean Altiplano. We also evaluate the physical and chemical changes of the lake over time by studying the mineralogy and geochemistry of the terrace profile.
Results: The mineralogy and geochemistry of the terrace profile revealed large water level fluctuations in the lake, scarcity of organic carbon, and high concentration of SO42--S, Na, Cl and Mg. Lipid biomarker analysis indicated the presence of aquatic/terrestrial plant remnants preserved in the ancient sediments, and genome-resolved metagenomics unveiled a diverse prokaryotic community with still active microorganisms based on in silico growth predictions. We reconstructed 591 bacterial and archaeal metagenome-assembled genomes (MAGs), of which 98.8% belonged to previously unreported species. The most abundant and widespread metabolisms among MAGs were the reduction and oxidation of S, N, As, and halogenated compounds, as well as aerobic CO oxidation, possibly as a key metabolic trait in the organic carbon-depleted sediments. The broad redox and CO2 fixation pathways among phylogenetically distant bacteria and archaea extended the knowledge of metabolic capacities to previously unknown taxa. For instance, we identified genomic potential for dissimilatory sulfate reduction in Bacteroidota and α- and γ-Proteobacteria, predicted an enzyme for ammonia oxidation in a novel Actinobacteriota, and predicted enzymes of the Calvin-Benson-Bassham cycle in Planctomycetota, Gemmatimonadota, and Nanoarchaeota.
Conclusions: The high number of novel bacterial and archaeal MAGs in the Laguna Lejía indicates the wide prokaryotic diversity discovered. In addition, the detection of genes in unexpected taxonomic groups has significant implications for the expansion of microorganisms involved in the biogeochemical cycles of carbon, nitrogen, and sulfur. Video Abstract.
Keywords: Ancient sediments; Andean Altiplano; Genome-resolved metagenomics; Lipid biomarkers; Mars; Microbial dark matter; Microbial metabolism.
© 2024. The Author(s).
Conflict of interest statement
The authors declare no competing interests.
Figures
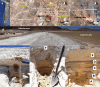
Study site and sampling area located in the Altiplano–Puna Plateau in the Central Andes. a and b show a sequential zoom of the location of Laguna Lejía (red pin) in northern Chile, where the Altiplano–Puna Volcanic Complex (APVC) is located. c shows the endorheic basin of Laguna Lejía surrounded by the active volcanoes Lascar and Simba. d shows the panoramic view of Laguna Lejía showing the terraces and the dry shore due to water retreat over the years. The white box indicates the location of the sampled sediment profile, located the first meter above the base of the terrace. e shows the ~1-m high vertical profile sampled. f and g zoom in the sampled profile and show the six sediment layers collected from the bottom (A) to top (F) based on their different color and texture. Starting from the first meter of the terrace, samples were collected from 1 to 1.07 m (A), 1.08 to 1.09 m (B), 1.10 to 1.35 m (C), 1.36 to 1.38 m (D), 1.39 to 1.70 m (E) and 1.71 to 1.95 m (F) high. Pictures a, b, and c are from Google Earth.
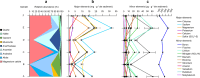
Mineralogical and geochemical composition of the six sediment samples from the Laguna Lejía terrace (10.3–11 kyr BP). a Mineralogical composition of the crystalline fraction measured by X-ray diffraction (XRD). The minerals with the blue color scale are feldspars. b Major and c minor elements in the soluble fraction of sediment samples. The sulfur concentration was calculated from sulfate, and the nitrogen concentration was calculated from nitrate since nitrite was below the quantification level (1.6 ppb). Error bars represent the standard deviation of triplicate measurements
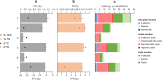
Lipid and bulk isotopic composition of the organic matter in the six sediment samples from the Laguna Lejía terrace. a Total organic carbon (TOC) and stable-carbon isotopic ratio (δ13C). b Total nitrogen (TN) and stable-nitrogen isotopic ratio (δ15N). TN concentration was not detected in samples B and D. c Composition of the three polarity fractions of lipids (non-polar, blue color shades; acidic, red color shades; and polar, green color shades). Error bars represent the standard deviation of triplicates. Error bars in δ13C are behind the dots and are smaller than the dots’ size
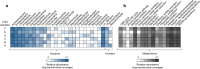
Prokaryotic community composition and metabolic potential of the Laguna Lejía terrace sediments (A to F). a Prokaryotic community composition at the phylum level (blue color scale) based on the rpS3 gene similarity to the GTDB database (r95). The heatmap is made by summing scaffold coverage containing the rpS3 gene, normalized by sequencing depth, summed by phylum and sample, and then log-transformed to help visualization of the less abundant phyla. b Prokaryotic metabolic potential (gray color scale) based on the prediction of key enzymes. The heatmap is made by summing scaffold coverage containing genes encoding for specific key enzymes, normalized by sequencing depth, summed by metabolism and sample, and then log-transformed to help visualization of the less abundant metabolisms. Key genes and enzymes involved in each specific metabolism are listed in Table S3. No enzymes were annotated to the 3-HP bi-cycle and N2 fixation. "Un." means unclassified
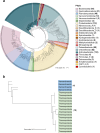
Maximum Likelihood unrooted phylogenies (model selected in IQ-TREE by Modelfinder) of the new a 572 bacterial genomes and b 19 archaeal genomes reconstructed from the Laguna Lejía terrace. Phylogenies were constructed using a concatenation of 30 ribosomal proteins. In brackets, the number of MAGs at the phylum level
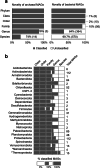
Novelty quantification of total bacterial and archaeal MAGs from the Laguna Lejía terrace. a Proportion of classified (gray) and unclassified (white) total archaeal and bacterial MAGs at different taxonomic levels using GTDB. In brackets, the number of unclassified MAGs. b Proportion of classified (gray) and unclassified (white) total archaeal (asterisks) and bacterial MAGs grouped by phylum at different taxonomic levels.
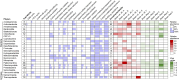
Genomic features, predicted metabolic potential, and replication (iRep) indices of 591 MAGs recovered from the Laguna Lejía terrace grouped by phylum. The completeness of purple bars within cells represents the abundance of total MAGs that have annotated at least one key enzyme for a specific metabolic pathway within a phylum. Key enzymes involved in each specific metabolism are listed in Table S3. No enzymes were annotated to the 3-HP bicycle and N2 fixation. Color gradients represent the mean relative abundances (red) or iRep values (green) of dereplicated MAGs for each phylum. The genomic features and predicted metabolic potential and replication indices of each individual MAG are shown in Table S7
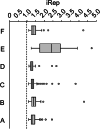
Predicted genome replication (iRep values) of bacterial MAGs from the Laguna Lejía terrace at the time of sampling. The dashed line represents the threshold above which population genomes are considered to be replicating. N in each sample is: 73 for A, 73 for B, 111 for C, 67 for D, 41 for E, and 73 for F
Similar articles
-
A metagenomics roadmap to the uncultured genome diversity in hypersaline soda lake sediments.
Vavourakis CD, Andrei AS, Mehrshad M, Ghai R, Sorokin DY, Muyzer G. Vavourakis CD, et al. Microbiome. 2018 Sep 19;6(1):168. doi: 10.1186/s40168-018-0548-7. Microbiome. 2018. PMID: 30231921 Free PMC article.
-
Parro V, Blanco Y, Puente-Sánchez F, Rivas LA, Moreno-Paz M, Echeverría A, Chong-Díaz G, Demergasso C, Cabrol NA. Parro V, et al. Astrobiology. 2018 May;18(5):586-606. doi: 10.1089/ast.2015.1342. Epub 2016 Nov 28. Astrobiology. 2018. PMID: 27893284
-
Abdallah RZ, Elbehery AHA, Ahmed SF, Ouf A, Malash MN, Liesack W, Siam R. Abdallah RZ, et al. mSystems. 2024 Jun 18;9(6):e0009524. doi: 10.1128/msystems.00095-24. Epub 2024 May 10. mSystems. 2024. PMID: 38727215 Free PMC article.
-
New Microbial Biodiversity in Marine Sediments.
Baker BJ, Appler KE, Gong X. Baker BJ, et al. Ann Rev Mar Sci. 2021 Jan;13:161-175. doi: 10.1146/annurev-marine-032020-014552. Epub 2020 Aug 3. Ann Rev Mar Sci. 2021. PMID: 32746696 Review.
-
Reyes-Umana V, Ewens SD, Meier DAO, Coates JD. Reyes-Umana V, et al. mBio. 2023 Dec 19;14(6):e0043123. doi: 10.1128/mbio.00431-23. Epub 2023 Oct 19. mBio. 2023. PMID: 37855625 Free PMC article. Review.
References
-
- Yarza P, Yilmaz P, Pruesse E, Glöckner FO, Ludwig W, Schleifer K, et al. Uniting the classification of cultured and uncultured bacteria and archaea using 16S rRNA gene sequences. Nat Rev Microbiol. 2014;12:635–45. - PubMed
MeSH terms
Substances
Grants and funding
- FJC2018-037246-I/Spanish Ministry of Science and Innovation/State Agency of Research
- RYC2018-023943-I/Spanish Ministry of Science and Innovation/State Agency of Research
- RYC-2014-19446/Spanish Ministry of Science and Innovation/State Agency of Research
- RTI2018-094368-B-I0/Spanish Ministry of Science and Innovation/State Agency of Research
- PEJD-2017- POST/TIC-4119/Spanish Ministry of Science and Innovation/State Agency of Research (EU Youth Employment Initiative )
LinkOut - more resources
Full Text Sources
Research Materials
Miscellaneous