Heme homeostasis and its regulation by hemoproteins in bacteria - PubMed
- ️Mon Jan 01 2024
Review
. 2024 Jul 11;3(3):327-342.
doi: 10.1002/mlf2.12120. eCollection 2024 Sep.
Affiliations
- PMID: 39359680
- PMCID: PMC11442138
- DOI: 10.1002/mlf2.12120
Review
Heme homeostasis and its regulation by hemoproteins in bacteria
Yingxi Li et al. mLife. 2024.
Abstract
Heme is an important cofactor and a regulatory molecule involved in various physiological processes in virtually all living cellular organisms, and it can also serve as the primary iron source for many bacteria, particularly pathogens. However, excess heme is cytotoxic to cells. In order to meet physiological needs while preventing deleterious effects, bacteria have evolved sophisticated cellular mechanisms to maintain heme homeostasis. Recent advances in technologies have shaped our understanding of the molecular mechanisms that govern the biological processes crucial to heme homeostasis, including synthesis, acquisition, utilization, degradation, trafficking, and efflux, as well as their regulation. Central to these mechanisms is the regulation of the heme, by the heme, and for the heme. In this review, we present state-of-the-art findings covering the biochemical, physiological, and structural characterization of important, newly identified hemoproteins/systems involved in heme homeostasis.
Keywords: heme; heme acquisition and utilization; heme export; heme homeostasis; hemoprotein.
© 2024 The Author(s). mLife published by John Wiley & Sons Australia, Ltd on behalf of Institute of Microbiology, Chinese Academy of Sciences.
Figures
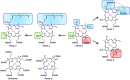
Chemical structures of hemes a, b, c, o, d, d 1, and siroheme. Heme b is the prototype heme species of all known heme synthetic pathways from which other “genuine” hemes are derived, including o, a, c, and d. Differences among them are highlighted in colored boxes. Siroheme and heme d 1 are not derivatives of heme b.
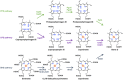
Heme biosynthetic pathways found in bacteria. The steps that are conserved in both eukaryotes and prokaryotes leading to the production of the common intermediate uroporphyrinogen III are not shown. From uroporphyrinogen III, heme biosynthesis in bacteria can be carried out through three distinct pathways: protoporphyrin‐dependent (PPD), coproporphyrin‐dependent (CPD), and siroheme‐dependent (SHD) pathway.
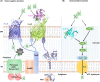
Schematic overview of heme acquisition in Gram‐negative bacteria and Gram‐positive bacteria. (A) Representative heme acquisition systems in Gram‐negative bacteria. The heme is shown as a green ball‐stick model. Heme translocation pathways across the outer membrane (OM) mediated by type I and type II TonB‐dependent receptors (TBDRs), represented by Pseudomonas aeruginosa PhuR and Serratia marcescens HasR (together with hemophore HasA), respectively (PDB ID: 5C58), are shown. The ABC transporter, composed of periplasmic SBP (a substrate‐binding protein), inner membrane (IM)‐bound permease, and cytoplasmic ATPase, is responsible for heme translocation across the IM. HasA/HasR also functions as a heme sensor for the HasS/HasI regulatory system. Upon activation, anti‐σ HasS releases σ HasI, allowing the latter to direct transcription of its target genes. (B) Iron‐regulated surface determinant (Isd) system for Gram‐positive bacteria heme acquisition in Staphylococcus aureus (IsdB, PDB ID: 3RUR).
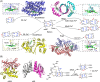
Heme degradation in bacteria. (A) Representative heme oxygenases from four distinct groups: Corynebacterium diphtheriae HmuO (PDB ID: 1IW0), Mycobacterium tuberculosis MhuD (PDB ID: 4NL5), Yersinia enterocolitica HemS (PDB ID: 2J0P), and Helicobacter pylori HugZ (PDB ID: 3GAS). The resultant products of heme degradation, biliverdin, mycobilin, or staphylobilin are shown in the chemical structures. (B) Heme c oxygenases. cHOA (PDB ID: 6VNA) and cHO were predicted using Colabfold. The heme is shown as a green ball‐stick model. The resultant products of heme degradation are shown in the chemical structures. cHOA, c‐type heme oxygenase A.
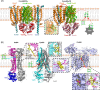
Heme exports in bacteria. The heme in the cytoplasm and the membrane is shown as a green ball‐stick model, while the transporter is shown as a yellow ball‐stick model. The transport routes of heme substantiated experimentally and proposed are shown by solid and dashed arrow lines, respectively. (A) Structures of HrtBA, CydDC, and CcsBA. Corynebacterium diphtheriae HrtBA is heterodimer (PDB ID: 7W7D), with one monomer in gray and the other in magenta and blue; Escherichia coli CydDC is heterodimer (PDB ID: 8IPS), with CydC in cyan and CydD in gray; Helicobacter hepaticus CcsBA is in open conformation (PDB ID: 7S9Y). Heme could be present at two sites. The surface image shows a side view of heme binding. (B) The CcmABCD system in two cryo‐EM structures (PDB ID: 8CE1, 7F04) with different complex compositions, Ccm(ABCD)2 or Ccm(AB)2CD. The CcmA subunits (red and raspberry), CcmB subunits (green and splitpea), CcmC (orange), and CcmD (deep teal) are shown. After synthesis, hemes may enter the membrane autonomously or with the help of the heme‐trafficking protein HtpA. CcmABCD translocates heme to CcmC from the cytoplasm, or likelier from the membrane.
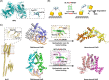
Regulatory hemoproteins containing Cys–Pro (CP) motifs and non‐CP motifs. (A) Representative hemoprotein with a CP motif for heme coordination, IRP2 (PDB ID: 6VCD). (B) Heme‐induced dissociation of Irr from the target gene and oxidative modification. (C) Representative hemoproteins with non‐CP motifs for heme coordination, including PerR, HrtR, and TCS HrrS. Heme‐induced dissociation of PefR and HrtR from the target genes are shown. The structures displayed on the top‐right, top‐left, bottom‐right, and bottom‐left corners, respectively, are Streptococcus agalactiae heme‐bound PefR (PDB ID: 7DVR), S. agalactiae DNA‐bound PefR (PDB ID: 7DVV), Lactococcus lactis heme‐bound HrtR (PDB ID: 3VP5), and L. lactis DNA‐bound HrtR (PDB ID: 3VOK). The structure of sensor kinase HrrS is predicted by AlphaFold2. Heme‐binding sites are shown in the close‐up view of the protein structure.
Similar articles
-
Overcoming the heme paradox: heme toxicity and tolerance in bacterial pathogens.
Anzaldi LL, Skaar EP. Anzaldi LL, et al. Infect Immun. 2010 Dec;78(12):4977-89. doi: 10.1128/IAI.00613-10. Epub 2010 Aug 2. Infect Immun. 2010. PMID: 20679437 Free PMC article. Review.
-
Ferrochelatase: Mapping the Intersection of Iron and Porphyrin Metabolism in the Mitochondria.
Obi CD, Bhuiyan T, Dailey HA, Medlock AE. Obi CD, et al. Front Cell Dev Biol. 2022 May 12;10:894591. doi: 10.3389/fcell.2022.894591. eCollection 2022. Front Cell Dev Biol. 2022. PMID: 35646904 Free PMC article. Review.
-
Heme Synthesis and Acquisition in Bacterial Pathogens.
Choby JE, Skaar EP. Choby JE, et al. J Mol Biol. 2016 Aug 28;428(17):3408-28. doi: 10.1016/j.jmb.2016.03.018. Epub 2016 Mar 24. J Mol Biol. 2016. PMID: 27019298 Free PMC article. Review.
-
The role of host heme in bacterial infection.
Donegan RK. Donegan RK. Biol Chem. 2022 Oct 14;403(11-12):1017-1029. doi: 10.1515/hsz-2022-0192. Print 2022 Nov 25. Biol Chem. 2022. PMID: 36228088 Review.
-
Heme Mobilization in Animals: A Metallolipid's Journey.
Reddi AR, Hamza I. Reddi AR, et al. Acc Chem Res. 2016 Jun 21;49(6):1104-10. doi: 10.1021/acs.accounts.5b00553. Epub 2016 Jun 2. Acc Chem Res. 2016. PMID: 27254265 Free PMC article. Review.
References
Publication types
LinkOut - more resources
Full Text Sources